Erika Kwong, Michel Ntiri, Anjali Patel, Liz Ramos, Aysha Rehman
Department of Biology, Rutgers University, Camden NJ 08102
Edited by Zachary Borden
Abstract
This study explores the effects of an anticoagulant on the blackworm, Lumbriculus variegatus, to substantiate the claim that L. variegatus can serve as a model organism for the human blood clotting process. This information could initiate further research for alternative and more efficient coagulative treatments for those who suffer from blood clotting disorders, such as hemophilia. This experiment evaluated the coagulative ability of the blackworm, Lumbriculus variegatus, in the presence of the blood thinner, enoxaparin, in order to simulate a clotting disorder. The data showed a direct relationship between the dosages of enoxaparin given to the cut specimens and the time required to complete blastema formation, which designates the end of coagulation. These data suggest that an increase in dosage of enoxaparin decreases the coagulation rate in L. variegatus. The study supports that L. variegatus can serve as a model organism, specifically in the process of clotting.
Introduction
The coagulation process in annelids is mechanistically similar to the plasma coagulation in mammals. In annelids, coagulation is mainly a plasmatic process that does not require the presence of coelomocytes (Valembois et al., 1988). Plasma coagulation in mammals converts soluble fibrinogen to insoluble fibrin, which is initiated by the cleavage of fibrinogen by the serine protease, thrombin. Comparatively, fetidin, a plasma protein in the annelid clotting pathway, is also converted from a soluble form to an insoluble form. In L. variegatus, thrombin-like serine proteases cleave fetidin to begin the coagulative process, which is analogous to the process of coagulation in humans (Tweeten and Reiner, 2012). Coagulation in L. variegatus is also stabilized by Factor XIII-like transamidases or SH-enzyme, which is related to Factor XIII administered to hemophiliacs (Valembois et al., 1988). Annelids are appropriate for this experiment because they can serve as simple, low-hazard models, which allows their known healing mechanisms to be researched extensively. These mechanisms can be used as analogues to the immunological responses of vertebrates and general human healing mechanisms (Bilej et al., 2013). Emulation of their natural habitat is simple and their average coagulation time is short enough to observe in one sitting within a lab environment on a low-grade stereomicroscope. If the low-hazard L. variegatus were proven to be viable model organisms, this would impact research and treatments for coagulation disorders. Worldwide, there are over 350,000 people with hemophilia and 280,000 of them (80%) do not receive treatment (Lee et al., 1998). Many patients with blood clotting diseases do not have access to proper treatment because of its cost, frequency, or technological accessibility. Furthermore, over 75% of those who suffer from bleeding disorders receive either inadequate or no treatment (Skinner, 2006). Current treatments for hemophilia involve administering the missing proteins in the blood via plasma containing clotting factors. The findings from this study reinforce that L. variegatus can be used as a model organism for human clotting, and can provide additional background information for further research, resulting in more accessible treatments for coagulation disorders such as hemophilia. This study analyzed the correlation between time of blastema formation and dosage of the anticoagulant, enoxaparin.
Materials and Methods
Preliminary analysis/care of L. variegatus: Samples of Lumbriculus variegatus used in all experiments were sourced from Dr. Shain at Rutgers University, Camden. They had been obtained locally from “Value Pet and Tropical Fish Center” pet store (Pennsauken, NJ). In the preliminary experiments #1 and #2, L. variegatus were kept in closed glass containers at room temperature (22° C), while preliminary experiment #3 and final experiment L. variegatus were kept at 10° C in 0.5% Instant Ocean solution until experiments were performed. The 0.5% Instant Ocean solution (IOS) consisted of 0.2 g NaCl in 1 L of sterile water at a pH of seven and was made in the lab as necessary (using autoclaved tap water and table salt). Three different preliminary experiments were performed to determine the rate of coagulation, the dosages needed for experimental trials, and the treatment method to establish the experimental procedure described below. Determination of Baseline Response: The first experiment determined the approximate time for a worm to coagulate as a baseline. Five specimens were put into individual petri dishes with fresh water and cut approximately in half with unused (not sterile) razor blades. The heads and tails were separated into individual petri dishes each with 20 mL fresh water, then examined and photographed under a stereomicroscope at twenty minute intervals for an hour in order to establish clear photographs, and note behavioral and physical changes. These specimens were observed at twenty minute intervals for an hour each day for four days (N = 5). The start and finish baselines of coagulation were established through daily pictures and observations using the stereomicroscope. Coagulation Determination: The specimens were observed and monitored under a stereomicroscope until the end of coagulation, denoted by the formation of a blastema (Fig. 1), or until 90 minutes had elapsed. Blastema formation marked the end of coagulation and therefore, was utilized as the baseline finish for this study (Truby, 1983). A blastema is a mass of undifferentiated cells that are capable of growth and regeneration into organs or body parts (Tweeten and Reiner, 2012). Blastema formation was defined by the circulation of blood of the dorsal blood vessel to the cut end of the specimen, the uniformity of color within the blastema region and the rest of the worm body, and a smooth rounded tip at the cut region. To establish a feasible procedure, experimental trials were stopped at a 90-minute maximum, which was twice the average time of coagulation. If trials surpassed this time limit, the subjects were deemed unable to coagulate; this may have offset the data.

Figure 1: Images of the same L. variegatus in 0.5% IOS, from left to right shows Time 0 after cut until blastema formation at 40 minutes. The last image shows the severed end rounded out, the dorsal blood vessel pumping blood to the tip of the cut end, and the color of the severed tip assimilating to the same hue as the rest of the worm’s body.
Determination of Safe Dosage: The second experiment determined which dosages of enoxaparin, an anticoagulant that inactivates thrombin and prevents thrombin from cleaving fibrinogen, would yield results without killing the specimens. The determination of the range of effective doses that would not kill the specimens was paramount for deciding the treatment dosage of enoxaparin in the final experiment. Enoxaparin sodium was obtained from Dr. Maria Choy, M.D., Central Jersey Neurological Institute, PA. The appropriate dosage of enoxaparin for one person is one 100 mg/mL syringe as one time use. This was further diluted in sterile IOS to be approximately proportionate to the average mass of L. variegatus (8 mg). One specimen remained uncut and submerged in IOS with 1 μL of enoxaparin as the control, and there was one cut specimen per dosage of enoxaparin for treatment iterations. Four aliquots: 0.1 μL, 1 μL, 10 μL, and 100 μL enoxaparin, were added to the initial 20 mL of IOS in each petri dish containing a cut worm. Specimens were observed under the dissecting microscope for physical changes of the cut region and behavioral changes. These observations were photographed every 30 minutes for an hour over a four-day period to determine the threshold dosage of enoxaparin.
Determination of Treatment Efficacy: The third experiment determined the exact experimental procedure and established the order in which the specimens were administered the blood thinner, cut, and photographed. The main focus of this preliminary examination was to decide whether to cut L. variegatus before exposing them to the treatment and whether to leave the specimens in the treatment after exposure. The three different dosages of enoxaparin in this experiment were 0.1 μL, 1 μL, and 10 μL of enoxaparin in 20 mL of IOS per petri dish. Four method types were tested over four days to find an optimal method that yielded the most consistent results. The first method involved submerging the specimens in salt water treated with one of the three aforementioned dosages of enoxaparin, cutting the specimens approximately in half while in the treatment, and then transferring the cut specimens into their isotonic natural salt water environment (20 mL IOS) after an hour under treatment. The second method involved cutting the specimens in a dry environment for more control when cutting and handling the specimens, submerging the cut specimen in treated salt water with one of the three dosages of enoxaparin, and then transferring the cut specimens into their natural salt water environment after an hour under treatment. The third method involved cutting the specimens first in a dry environment, then submerging them in treated salt water with one of the three dosages of enoxaparin, and observing them while still in the treated environment. The fourth method involved submerging the specimens in treated salt water with one of the three dosages of enoxaparin, cutting them while in treatment, and then observing them in treatment. For each of these methods, L. variegatus was observed under the stereomicroscope for approximately two hours per day. Observers noted the physical growth of the cut region in each specimen, each specimen’s behavior, and the duration of the coagulative process to best determine which treatment method was consistently effective. Each day, one of the four methods was tested on 16 specimens, for a total of 64 specimens over the four-day experiment. The 16 specimens were divided into groups of four. The first group was cut in the petri dish containing 20 mL IOS, serving as the positive control. Specimens in groups 2, 3, and 4 were treated with 0.1 μL enoxaparin in 20 mL IOS (minimum dosage), 1 μL enoxaparin per 20 mL IOS (median dosage), or 10 μL enoxaparin per 20 mL IOS (maximum dosage), respectively. The syringe dosage (100 mg/mL) was diluted to make stock solutions of 50 μL enoxaparin, 500 μL enoxaparin, 5000 μL enoxaparin, and 50,000 μL enoxaparin per 1 L sterile IOS stock solution for use in the third preliminary experiment and final experiment.
Experimental Protocol: The exact protocol used was determined from the third preliminary examination. The specimen was cut on a separate new plate, submerged into a given treatment, and observed while in treatment. Worms were observed under a stereomicroscope, and the time for blastema formation was recorded. Observations for each specimen were halted at the time of blastema formation or after 90 minutes if there was no blastema formation; this affected the data. Specimens were individually separated into petri dishes for experimental trials, while unused worms were kept in an incubator at 10°C. There were two controls and one experimental variable tested across three different dosages of enoxaparin in IOS. The positive control consisted of the head portion of a bisected worm in 20 mL IOS, and the negative control consisted of an uncut worm in 20 mL of enoxaparin solution at a dosage of 0.1 μL enoxaparin per 20 mL IOS. For each treatment, there were three replicates in individual petri dishes where only the cut heads were observed and the cut tails of bisected specimens were disposed. For the positive control, three specimens were cut on a dry petri dish lid (although plastic pipettes with IOS were used to transfer the specimens), and then placed in their respective sterile petri dishes containing a 20 mL IOS using a sterile plastic pipette. For the negative control, three other specimens were placed (uncut) in the minimum dosage of enoxaparin (0.1 μL enoxaparin/20 mL IOS). For the experimental variable, enoxaparin was diluted and separated into three distinct doses (0.1 μL, 1 μL, and 10 μL enoxaparin/20 mL IOS), creating three different experimental iterations. One person was assigned to one replicate which consisted of continuous observations of one positive control specimen, one negative control specimen, one minimum dosage specimen, one median dosage specimen, and one maximum dosage specimen. A given trial had three replicates, where each specimen was placed in a dry petri dish lid, cut at the same time (stopwatch recorded time), and then only its head was transferred to the petri dish containing blood thinner treatment while the tail was discarded (with exception to the negative control specimen which was left uncut). The petri dishes were then transferred to the respective workbenches for continuous observations under a stereomicroscope (one stereomicroscope per replicate). The heads were observed continuously and concurrently in one replicate, while any physiological changes were noted. Time to reach a fully formed blastema was observed under the stereomicroscope and recorded.
Statistical analysis: To first test the assumptions of normality and homogeneity of variance, Shapiro-Wilks and Bartlett tests were performed on the data using R. A significance analysis using student’s t-test was done on Microsoft Excel and R. Data was averaged for each of the variables in the experiment in order to deduce the significance in relation to the control.
Results
In all three trials, the average rate of coagulation of worms across doses was about 45 minutes. In trial 1, the average rate of coagulation of L. variegatus (in fresh water) was 40 minutes. In trial 2, the highest dosage (100 μL enoxaparin/20 mL IOS) consistently killed the heads and tails of all replicates within 20 – 48 hrs after the initial cut. The doses 0.1 μL enoxaparin through 10 μL enoxaparin per 20 mL IOS had more heads and tails that lived than those that died for the duration of the trial (four days). Trial 3 determined the most consistent and reliable method to administer enoxaparin to L. variegatus was to cut the specimen, submerge it into the treated environment, and keep it in the treated environment for observations for 2 hrs. The experimental results showed a direct relationship between dosage of enoxaparin in IOS and time of blastema formation; t-test, α=0.05, minimum dosage p=0.205, median dosage p= 0.016 and maximum dosage p=0.011. There was a general increase in coagulation time as the minimum dosage of the blood thinner increased; logarithmic regression: slope=0.0521, r2 = 0.92324 (Figure 2).
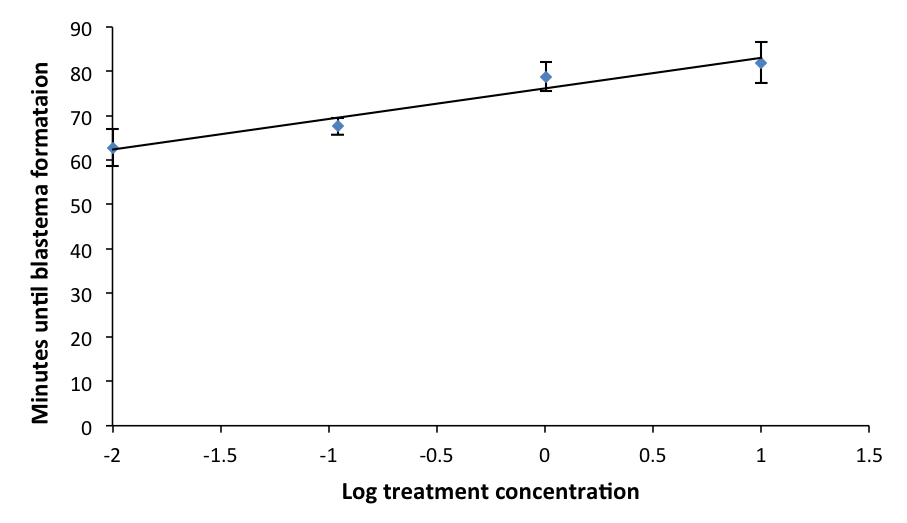
Figure 2: Mean time of blastema formation in L. variegatus across different dosages. Values for x-axis were log transformed from initial dosage treatments of 0µL, 0.1 µL, 1 µL, and 10 µL. Before log transformation, 0.01 was added to each due to one value being 0. Standard error is indicated.
Discussion
This study supports the assertion that annelids are appropriate comparative models for studying coagulation in mammals due to the mechanistic similarities in their responses to trauma (Bilej et al., 2013). There are significant similarities between the coelomic fluid composition of L. variegatus and the treatments administered to hemophiliacs. Plasma comprises a significant portion of treatment injections and the coelomic fluid of L. variegatus behaves similarly to human plasma. Generally, as the dosage of enoxaparin increased, the time for blastema formation also increased (Fig. 2). Because blastema formation marks the end of coagulation, this demonstrates the direct relationship between dosage of enoxaparin and time of coagulation. The data show that increased dosages of enoxaparin slowed down the rate of coagulation in L. variegatus. Out of the three dosages tested, the data for the control compared to both the maximum and median doses individually were statistically significant, meaning that these two doses showed a significant effect on the rate of coagulation in L. variegatus. The average times between the control and minimum dosage was not statistically significant. Therefore, the dosage of blood thinner administered to the control group and to the minimum group did not have a significant effect on the coagulation rate. When the results from the median and maximum dosages were compared to the minimum individually, both data sets showed statistical significance. The data for the median and maximum dosages did not show statistical significance when compared to each other. However, this could be due to the fact that any observations that exceeded the 90 min mark were recorded as 90 min, and most of the specimens that surpassed the 90 min mark were in the median or maximum treatments. This strengthens the conclusion that there is a direct relationship between concentration of enoxaparin and the time for blastema formation. The capability of the anticoagulant to slow down the normal coagulation process in L. variegatus became clearly apparent as dosages were increased, demonstrating how these specimens respond to an environment simulating a blood clotting disorder. Since clotting factors in both annelids and mammals are mediated by serine proteases, this experiment could serve as a platform to further clotting research for more efficient treatments and improve accessibility. Further similarities between L. variegatus and humans would be necessary to facilitate further research regarding these factors and eventually improve blood clotting treatment. Functional analysis would be critical to determine the physiological similarities between the clotting factors in L. variegatus and those in humans. Genomes in L. variegatus have not been sequenced yet. Therefore, further research could be implemented in order to study the sequence or functional similarities of the genes involved in coagulation in humans and in L. variegatus. Additionally, one could study anticoagulants in a different drug classes because enoxaparin is in the heparin class, which uses a distinctive mechanism of action compared to the other classes. These suggested studies in the future may support that L. variegatus could be a model organism for understanding the human coagulation process, and also for screening a useful drug in blood coagulation processes.
Acknowledgements
Special thanks to Dr. Kwangwon Lee and Zachary Borden for overseeing the study, Dr. Amy Savage for supplying three of her dissecting microscopes, Dr. Daniel Shain and Ralph Saunders for supplying the L. variegatus and advice, Professor Tracie Paulson for her advice, Josh Waters for assisting the lab group and ensuring their safety, and the Rutgers Camden Biology Department for supporting the study.
References
Bilej, M., Procházková, P., Šilerová, M., and Josková, R. (2010). Earthworm Immunity. In: Soderhall, L. (Ed.), Invertebrate Immunity Advances in Experimental Medicine and Biology, vol. 708, pp. 64-67.
Lee, C.A., Kessler, C.M., Varon, D., Martinowitz, U., Heim, M., and Lee, C.A. (1998). Towards achieving global haemophilia care — World Federation of Hemophilia programmes. Haemophilia 4, 463–473.
Skinner, M.W. (2006). Treatment for all: a vision for the future. Haemophilia 12, 169–173.
Truby, P.R. (1983). Blastema formation and cell division during cockroach limb regeneration. Development 75, 151–164.
Tweeten, K.A., and Reiner, A. (2012). Characterization of serine proteases of Lumbriculus variegatus and their role in regeneration. Invertebr. Biol. 131, 322–332.
Valembois, P., Roch, P., and Lassegues, M. (1988). Evidence of plasma clotting system in earthworms. J. Invertebr. Pathol. 51, 221–228.
Journal of Biological Sciences at Rutgers Camden (JBS) is licensed under a Creative Commons Attribution-NonCommercial-ShareAlike 4.0 International License