Abstract
Introduction
A large portion of the population in the United States suffer from chronic pain (Dahlhamer et al., 2018). However, the mechanisms underlying chronic pain are still largely unknown. Treatments presently used to address chronic pain include chiropractic movements, nonsteroidal anti-inflammatory drugs, and opioids (Tompkins et al., 2017). However, these treatments are temporary and only treat the pain, rather than cure it. Opioids are one of the most widely prescribed treatments for chronic pain by clinicians; however, they are known to lead to addiction (Kosten and George, 2002). To better understand and treat chronic pain, the underlying mechanisms need to be identified and further studied.
Many foods commonly consumed in the United States are high in fat such as pizza, hamburgers, and french fries. Foods that are high in fat are more calorie dense compared to foods with high concentrations of other macronutrients, such as carbohydrates or proteins (Rolls, 2000). Therefore, individuals who consume high fat diets are more likely to consume higher calories compared to individuals who eat a balanced diet (Golay and Bobbioni, 1997). If the excess calories consumed are not used, the body will store them as adipose tissue, or fat. The storing of adipose tissue from a constant supply of surplus calories will eventually lead to an individual becoming overweight and ultimately obese. However, the pathology of obesity can stem from genetic disorders, hormonal imbalances, and dysregulation of glucose metabolism as well (Redinger, 2007). The body mass index (BMI) is used to determine if an individual is overweight or obese (Nuttall, 2015). However, the BMI system has limitations taking into account an individual’s muscle mass which would increase their BMI. Overweight in terms of the BMI is defined as 25 or more and obese is defined as 30 or more. Obesity was thought to lead to chronic pain solely through the excess stress it puts on the body (McVinnie, 2013). Now it is known that obesity puts an individual’s body in a pro-inflammatory state which is caused by the release of chemokines and cytokines from adipose tissue (Wang and He, 2018). Pro-inflammatory cytokines are released from certain immune cells to create an inflammatory response while chemokines are used to signal to other immune cells to migrate to the inflamed area.
One mechanism in which a high fat diet can increase pain sensitivity is through the immune system. The immune system can be affected by individuals’ dietary choices. A high fat diet has been found to elicit an immune response in which macrophages buildup in adipose tissue (Weisberg et al., 2003). This buildup of macrophages in adipose tissue can lead to inflammation and inflammation has been found to increase pain behaviors in rats regardless of obesity (Song et al., 2017). Pain behaviors consist of the actions and postural changes that occur after the experience of pain. These behaviors become increasingly exaggerated when more pain is experienced leading to the conclusion that individuals with a lower nociceptive sensitivity threshold will display increasing pain behaviors (Deuis et al., 2017).
D. melanogaster is the most used and one of the best understood model organisms in research (Tolwinski, 2017). They make excellent model organisms to study because they are very cheap and easy to maintain. D. melanogaster are also easier to study than vertebrates because they possess far fewer genes than other models (Im and Galko, 2012). While D. melanogaster have a much simpler genome than other model organisms, they hold many similarities with that of humans. Many genes that encode human diseases can similarly be found in D. melanogaster (Fortini et al., 2000). However, differences between cellular processes and physiology creates limitations translating findings into human models (Pandey and Nichols, 2011). Their short life cycle allows for repeated tests to be performed on new flies and larvae. D. melanogaster is a commonly studied model in the realm of pain research as there are a plethora of significant benefits to studying pain in this model organism. If a correlation is found between a high fat diet and pain response in this organism, then more research laboratories that do not have access to vertebrate models will have access to an invertebrate model organism to test pain response quickly and affordably. Nociception, or the nervous system’s response to dangerous stimuli, can be studied in a variety of ways including through the utilization of mechanical, thermal, and chemical assays. When exposed to a noxious stimuli, D. melanogaster larvae exhibit a “corkscrew rolling” behavior that can be easily identified and observed with the naked eye and recorded with a simple recording device such as a phone (Im and Galko, 2012). Many studies have looked at the effects of high fat diets on pain behaviors in vertebrate models (Benoit et al., 2013; Song et al., 2017, 2018). Invertebrate models, particularly D. melanogaster, have not been studied to determine the effects of a high fat diet on pain sensitivity. Previous research shows evidence that the CG14446 gene in D. melanogaster (responsible for feeling pain) shows a stronger trend to be triggered by a low protein to sugar diet ratio, but neither this gene nor the pain response have been tested against a high fat diet in the same organism (Jaime et al., 2017). By using D. melanogaster the effects of a high fat diet on pain sensitivity can easily be studied.
In this study, the effects of a high fat diet will be explored on pain sensitivity in an invertebrate model. We hypothesized that larvae consuming a high fat diet will experience a decrease in pain response latency when exposed to a noxious stimulus as demonstrated in other animal models. A possible mechanism in which a high fat diet is increasing pain sensitivity is through the immune system. A high fat diet has been shown to increase Unpaired-3 which is a pro-inflammatory cytokine in D. melanogaster (Woodcock et al., 2015). The increase in pro-inflammatory cytokines may be responsible for the increase in pain sensitivity when consuming a diet high in fat. To test this hypothesis, we separated flies into two groups, a high fat diet (HFD) and a regular diet (RD). Third instar larvae were collected from each vial and a chemical nociception assay was used to determine pain response latency.
Materials & Methods
Larvae Collection
Larvae of D. melanogaster grows in three stages after hatching from the embryo: first instar, second instar, and third instar—with the first two stages lasting approximately one day each and the third taking around two days (at 25 ℃). Third instar larvae can easily be identified because they can be seen crawling on the side of the tubes and are larger than first and second instar stages. However, because of the HFD tube’s slightly sticky conditions the third instar larvae were mostly found still inside of the food. To collect larvae for the experiment, the food needed to be taken out of the vial and the larvae had to be searched for by sifting through the food. Therefore, because larvae could not be seen on the side of the tube to be considered third instar, so they needed to be 3 to 4 millimeters in length. The larvae were collected at 3:00 pm eastern time on the day of each experiment. For each experiment, ten third instar larvae were collected from both the HFD and RD groups and placed on separate petri dishes. The wild-type flies utilized to produce the larvae were provided by Dr. Kwangon Lee’s and from the Bloomington Drosophila Stock Center.
Food Production
The RD was prepared by mixing equal volumes of dry fly food mix and distilled water. The dry fly food mix utilized Ward’s Instant Drosophila Medium (ingredients listed in Table 1). The food was then placed into a vial and promptly labeled RD. The HFD was prepared by mixing the same dry fly food mix with distilled water in equal parts by volume. Afterwards, 365 Everyday Value brand organic coconut oil was heated to the point of liquidization in a microwave safe dish for about 30 seconds in a 1100 W microwave. The coconut oil and fly food were then thoroughly mixed in a 3:7 part ratio by weight, resulting in a diet that is roughly 70% calories from fat. The food was then placed into a fresh vial and promptly labeled HFD. Following up, the sides were wiped clean to clear residue that might have stuck, so as to prevent the flies from sticking to the sides (Diop et. al., 2017).
Table 1. High Fat Diet Nutritional Information
Ingredients |
Amount Per Volume (g) |
Fat (g) |
Carbohydrates (g) |
Protein (g) |
Flour |
0.166 |
0.002 |
0.127 |
0.017 |
Baby Cereal |
0.296 |
0.02 |
0.198 |
0.04 |
Potato Flakes |
2.757 |
0 |
2.298 |
0.153 |
Base |
0.28 |
0.014 |
0.112 |
0.046 |
Water |
3.5 |
0 |
0 |
0 |
Coconut Oil |
3 |
3 |
0 |
0 |
Total Volume (g) |
10 |
3.036 |
2.735 |
0.256 |
Calories (kcal) |
39.288 |
27.324 |
10.94 |
1.024 |
Calorie % |
100% |
69.54% |
27.85% |
2.61% |
Fly Maintenance
The control group flies were placed into a vial containing RD. The vial was then placed on its side and set aside to allow the flies to reproduce. A second vial for the experimental group was prepared by depositing a mixture of fly-food and coconut oil at the bottom of the vial. The sides of the tubes were then wiped to remove any residue from the food mixture and then the flies were transferred inside. The vial was then placed horizontally so that the flies have a surface to rest on that is free of the food and must be maintained at a few degrees cooler than room temperature, so the coconut oil does not melt and trap the flies.
Pain Sensitivity Assay (PSA)
D. melanogaster were divided into two plastic Carolina brand vials: one of the vials contained RD while the other contained HFD. The RD served as the control while the HFD served as the experimental treatment group; after the flies were placed in the respective vials, the vials were promptly sealed with one cotton ball each. Once the flies mated and produced third instar larvae, the larvae were removed from the vials and isolated onto a Carolina brand polystyrene petri dish whereby 1.5 µl of 9% HCl (that was manually diluted from a more concentrated solution) was dropped onto them from a height of 1 cm using a Ward’s® Adjustable Volume Micropipette while being recorded with a recording device. They were then recorded until they exhibited a “corkscrew” rolling motion indicating their pain response (Im and Galko, 2012). Later, the video recordings were closely examined by the researchers to measure the latency time of the pain response. A visual of the experimental setup and method can be seen in Figure 1 as depicted using Biorender.com software tools to create the graphic.
The experiment consisted of larval groups from each diet containing 10 larvae each. One trial included testing a group of RD larvae, then a group of HFD larvae. Experiments were then repeated for a total of 3 sets of trials, or 60 larvae (30 RD larvae, and 30 HFD larvae) in total.
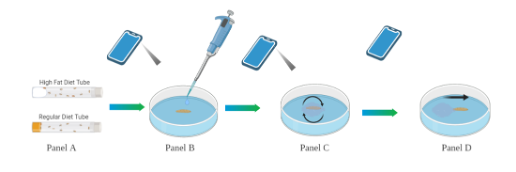
Figure 1. Pain Sensitivity Assay. Flies in the tubes (A), 3rd instar larvae are placed onto a petri dish in sight of the recording device (B), pain sensitivity assay (C), recording stopped after larvae exhibit sensitivity (D).
Results
To test if the HFD affects pain sensitivity, we performed PSA (Materials and Methods). After the completion of three trials, the average response latency for the ten larvae in both the HFD and RD groups were calculated. For the HFD groups, the average response latency(s) of the ten larvae were 3.1s, 4.4s, and 2.6s for Trials 1, 2, and 3 respectively. In the same manner, the average response latency(s) for the RD groups were calculated to be 5.375s, 4.8s, and 5.3s. Our data shows that the HFD group exhibited a lower average response latency in comparison to the RD group, two-tailed t-test, p = 0.001 (Fig. 2).
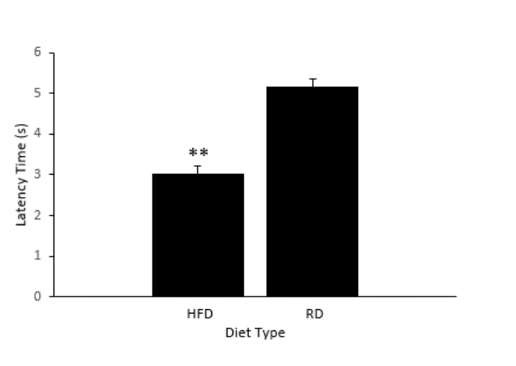
Figure 2. Latency times between diet types. HFD; High Fat Diet. RD; Regular Diet. There is a statistical difference between HFD and RD, t-test p = 0.001.
Discussion
Our data suggests that there is a statistical difference in pain sensitivity caused by the diet (Fig. 2). As the length of the latency period and sensitivity to pain are shown to have a negative correlation, it is then implied that the larvae in the HFD group had a higher sensitivity to pain. Due to the limitations of technology available in this experiment, the levels of pro-inflammatory cytokines were not able to be evaluated in each of the groups’ larvae. In future studies, serum cytokine levels should be taken and compared from both groups. If differences are found in the groups, the groups should be treated with nonsteroidal anti-inflammatory drugs and tested using the same chemical nociception assay. The group’s serum cytokine levels should also be reexamined after administering the nonsteroidal anti-inflammatory drugs.
Next, the limitations of time and resources impeded the investigation of what specifically caused the increase in pain sensitivity. This means that the HFD group may have shown a higher pain sensitivity due to other reasons outside of the investigation of the experiment. However, other studies conducted on the diet-pain relationship in vertebrates have indicated that the inflammation caused by a HFD increased an organism’s pain sensitivity. As such, the HFD groups shortened latency period can be attributed to their diet (Song et al., 2017, 2018). Although our findings did not eliminate the possibility that components of coconut oil induced the effects, prior research in other model organisms strongly suggest the response was caused by the high fat diet. Further studies would include controls that would negate the effect caused by the components of coconut oil. As previously mentioned, coconut oil has been utilized in other studies to serve as the base for a high fat diet in D. melanogaster models. As with any research study, the number of confounding variables must be minimized and while these particular issues did not have a substantial effect, they must be avoided if a future study is conducted.
As previously mentioned, D. melanogaster serves as an excellent model for scientific research due to the simplicity of their physical responses and the efficiency of their use. With the results garnered from this experiment, the relationship between high fat diet and pain sensitivity in D. melanogaster has shown potential for future studies that can further explore the relationship between pain and diet. In turn, these studies can be translated to the more pressing issues of chronic pain in humans and the underlying mechanisms caused by a high fat diet.
Acknowledgements
We would like to thank Dr. Nathan Fried and Dr. Kwangwon Lee for the support, guidance, and expert opinions they provided throughout the duration of the research. Additionally, we would like to thank Dr. Rawia Khasawneh for her assistance in determining the macronutrient concentrations of the unique fly-food diet used in this experiment. Finally, we would like to acknowledge Jenny Pan for providing inspiration in creating Figure 1.
References
Benoit, B., Plaisancié, P., Awada, M., Géloën, A., Estienne, M., Capel, F., Malpuech-Brugère, C., Debard, C., Pesenti, S., Morio, B., et al. (2013). High-fat diet action on adiposity, inflammation, and insulin sensitivity depends on the control low-fat diet. Nutr. Res. 33, 952–960.
Dahlhamer, J., Lucas, J., Zelaya, C., Nahin, R., Mackey, S., DeBar, L., Kerns, R., Von Korff, M., Porter, L., and Helmick, C. (2018). Prevalence of Chronic Pain and High-Impact Chronic Pain Among Adults — United States, 2016. MMWR Morb. Mortal. Wkly. Rep. 67, 1001–1006.
Deuis, J.R., Dvorakova, L.S., and Vetter, I. (2017). Methods Used to Evaluate Pain Behaviors in Rodents. Front. Mol. Neurosci. 10.
Diop, S.B., Birse, R.T., and Bodmer, R. (2017). High Fat Diet Feeding and High Throughput Triacylglyceride Assay in Drosophila Melanogaster. J. Vis. Exp. JoVE.
Golay, A., and Bobbioni, E. (1997). The role of dietary fat in obesity. Int. J. Obes. Relat. Metab. Disord. J. Int. Assoc. Study Obes. 21 Suppl 3, S2-11.
Im, S.H., and Galko, M.J. (2012). Pokes, sunburn, and hot sauce: Drosophila as an emerging model for the biology of nociception. Dev. Dyn. 241, 16–26.
Jaime, M.D.L.A., Hurtado, J., Loustalot-Laclette, M.R., Oliver, B., and Markow, T. (2017). Exploring Effects of Sex and Diet on Drosophila melanogaster Head Gene Expression. J. Genomics 5, 128–131.
Kosten, T.R., and George, T.P. (2002). The Neurobiology of Opioid Dependence: Implications for Treatment. Sci. Pract. Perspect. 1, 13–20.
McVinnie, D.S. (2013). Obesity and pain. Br. J. Pain 7, 163–170.
Song, Z., Xie, W., Chen, S., Strong, J.A., Print, M.S., Wang, J.I., Shareef, A.F., Ulrich-Lai, Y.M., and Zhang, J.-M. (2017). High-fat diet increases pain behaviors in rats with or without obesity. Sci. Rep. 7.
Song, Z., Xie, W., Strong, J.A., Berta, T., Ulrich-Lai, Y.M., Guo, Q., and Zhang, J.-M. (2018). High-fat diet exacerbates postoperative pain and inflammation in a sex-dependent manner. Pain 159, 1731–1741.
Stefan, P. The Fundamentals of Flying: Simple and Inexpensive Strategies for Employing Drosophila Genetics in Neuroscience Teaching Laboratories. 10.
Valenza, A., Bonfanti, C., Pasini, M.E., and Bellosta, P. (2018). Anthocyanins Function as Anti-Inflammatory Agents in a Drosophila Model for Adipose Tissue Macrophage Infiltration.
Wang, T., and He, C. (2018). Pro-inflammatory cytokines: The link between obesity and osteoarthritis. Cytokine Growth Factor Rev. 44, 38–50.
Weisberg, S.P., McCann, D., Desai, M., Rosenbaum, M., Leibel, R.L., and Ferrante, A.W. (2003). Obesity is associated with macrophage accumulation in adipose tissue. J. Clin. Invest. 112, 1796–1808.
Journal of Biological Sciences at Rutgers Camden (JBS) is licensed under a Creative Commons Attribution-NonCommercial-ShareAlike 4.0 International License