Shannon Cline, Cynthia Nieves, Aubrey Otis, Michelle McCarthy, Zoe Mickens Department of Biology, Rutgers University, Camden, NJ 08102 Edited by Daniel Pinolini
Abstract
Trimethylamine N-oxide (TMAO) is an amine oxide metabolite found at higher levels in humans that are experiencing cardiovascular stress. On the contrary, deep-sea fish produce higher levels of TMAO to counteract hydrostatic pressure. This compound is naturally produced in the nematode species Caenorhabditis elegans; however, its function has not been extensively studied. In this study, it was hypothesized that the presence of exogenous TMAO would increase thermal tolerance of C. elegans. The survival rate of C. elegans was measured under a constant thermal stress while being exposed to various serial dilutions of exogenous TMAO. It was found that the 3M dilution demonstrated an increase in the survival rate while 6M treatments decreased the survival rate in a 30°C environment. These results suggest there are optimal concentrations that can either increase or decrease the survival rate in high temperature environments.
Introduction
In humans, carnitine and choline are converted into the metabolite trimethylamine. These compounds are found in red meat, fish, eggs, and dairy (Van Hecke et al. 2016). Trimethylamine is subsequently oxidized and converted to the metabolite trimethylamine N-oxide (TMAO) by the protein flavin-containing monooxygenase isoenzyme FMO-3 (Yeung et al. 2007). When present in high concentrations, TMAO increases the risk of cardiovascular disease, thrombosis, and kidney failure. This trimethylamine conversion also occurs within deep-sea fish, however, higher levels of TMAO are in this case beneficial and function to counteract hydrostatic pressure (Bockus et al. 2016). Another member of the flavin-containing monooxygenase isoenzyme family, FMO-4, assumes a vital role in osmoregulation in C. elegans by encouraging excess water clearance in hypotonic conditions. Trimethylamine N-oxide is thought to be a product of this process (Hirani et al. 2016). Although TMAO is naturally occurring in C. elegans (Atherton et al. 2008), its function and production in the nematode is not fully understood. Due to its brief life cycle and rapid reproductive capabilities, C. elegans was chosen for this study as the ideal model organism to observe behavioral changes in stressful environments. Stressors that induce a response in worm include overcrowding, starvation, oxidative stress, heavy-metal stress, and thermal stress (Zhou et al. 2011). While under thermal stress, heat shock transcription factor (HSF) regulates heat shock inducible gene expression, which prevents cellular degeneration, thus playing a role in development, immunity, and aging in C. elegans (Morton et al. 2013). In C. elegans, the HSF homolog hsf-1 is responsible for both stress resistance and the stress-induced extension of lifespan. In high-temperature conditions, up-regulation of heat-shock proteins HSP-4 and HSP-16 occurs within the roundworm as a stress response (Zhou et al. 2011, Lithgow et al. 1994). These proteins may function to extend the longevity of C. elegans when in stressful environments by maintaining cellular proteostasis and preventing protein and cellular damage (Rodriguez et al. 2013). The purpose of this study was to investigate whether the presence of exogenous TMAO would affect the thermotolerance of C. elegans, and if so, determine whether TMAO serves to increase or decrease the survival rate. For the purposes of this study, worms in the L4 stage of their life cycle were used, as this is the stage prior to maturation into gravid adult. Response to stress was measured as the survival rate of the worms, as survival rate is known to be indicative of the thermotolerance of C. elegans (Zhou et al. 2011, Lithgow et al. 1994).
Materials & Methods
Care of C. elegans: The N2 wild type strain of Caenorhabditis elegans, acquired from CGC Stock center, was chosen for this research and kept in the Waterfront Technology Center in Camden, NJ. In accordance with the protocols described in the article by Brenner, they were maintained on Nematode Growth Medium (NGM) agar plates containing the uracil auxotroph E. coli strain OP50 as a source of nutrition. The plates containing the worms were placed in an incubator set to 20°C, the temperature in which they thrive. The worms were transferred to new plates when necessary to promote reproduction. In order to transfer the worms, the plates were washed with an M9 buffer to lift and move the worms to the edge of the plate. The M9 buffer containing the worms was then extracted from the edge of the plates into a single falcon tube, and centrifuged to form a worm pellet. After a worm pellet was formed, the nematodes were then re-suspended and transferred to a set of fresh NGM agar plates (Brenner, 1974). Egg Preparation In order to ensure that all of the worms were in the same developmental stage during the experimental trial, it was necessary to isolate the embryos by means of an egg preparation. To do this, a hypochlorite solution was used to break down the cuticles of worms while keeping the eggs intact. The eggs hatched into L1 larvae after 14-16 hours of rocking, and were then transferred to new NGM plates. Experimental Protocol Synchronized L1 worms were transferred to NGM plates containing OP50, or plates with various dilutions of TMAO mixed into the OP50 suspension. A sample egg preparation was performed to determine the time required for the worms to mature to the L4 stage. In trial one, 15 plates of worms were used. There were three replicate plates for each of the four different dilutions (0.75M, 1.5M, 3M, 6M), and three replicate plates of the control. After 45-47 hours, the worms developed into the L4 stage. The plates were then incubated at 30°C for the duration of the trial. The number of worms alive on each plate was counted initially, and then again every 2 hours over a 12-hour period. For the following two trials, the 0.75M and 1.5M dilutions were omitted due to the lack of a statistically significant difference in survival rate compared to the control. For trials two and three, 9 plates were used: three replicates of control plates, 3M TMAO plates, and 6M TMAO plates. Trials two and three were also completed over a 12 hour period. Trial two plates were incubated at 35°C, while trial three plates were incubated at 40°C. Active worms were carefully counted under the microscope before being placed under thermal stress, and then again every 2 hours. Survival was scored by mobility, and worms in a pin-like position were identified as dead.
Results
In the first trial when synchronized L4 worms were exposed to constant 30°C, the average percent of survival for the C. elegans that were not exposed to TMAO was 31.81%, while the worms treated with 6M TMAO had a 15.18% survival rate, and the worms treated with 3M had a 51.05% survival rate (Figure 1a). The average survival rate after eight hours for the control was 31.19%, that of the 3M was 64.81%, and that of the 6M was 15.28% (Figure 2). The survival rate of the worms treated with 0.75M TMAO (19.6% survival) and 1.5M TMAO (24.6% survival rate) did not show a significant difference from the control; therefore, these two concentrations were omitted from the final data. In the second trial, the average percent of survival after 12 hours of heat exposure at 35°C for the C. elegans exposed to no TMAO was 5.17%, while the worms exposed to 3M TMAO had a survival rate of 4.12%, and the worms exposed to 6M TMAO had a survival rate of 16.3% (Figure 1b). Plate two of the 3M dilution and plate three of the 6M had a 0% survival rate after the two-hour mark. After omitting these plates from the data, a Shapiro-Wilks test confirmed that the data was normally distributed, with a p-value of 0.6864. A two-way ANOVA test resulted in a p-value of 0.00843, which confirmed that the concentration of TMAO affected the survival rate depending on the temperature. The average survival rate after eight hours for the control was 42.29%, that of the 3M was 8.27%, and that of the 6M was 21.64% (Figure 2). The final trial tested the worms at 40°C. Every replicate of nematodes showed 0% survival rate throughout the 12 hour trial period.
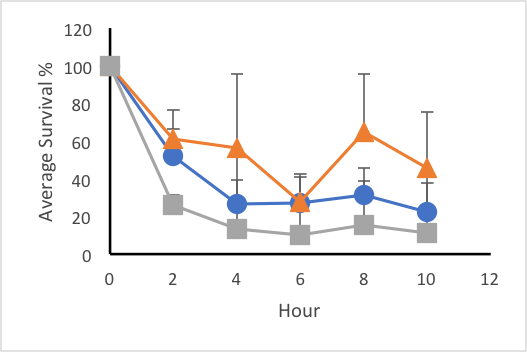
Figure 1: Effects of trimethylamine N-oxide on survival rate of C. elegans under constant thermal stress. Average survival rates of control (blue, circle), 3M solution treatment (orange, triangle), and 6M treatment (grey, square) groups over 12 hours at 30°C (a) and 35°C (b). Error bars represent standard deviation.

Figure 2: Average survival percentage of control after eight hours (blue, dotted), 3M solution treatment (orange, slanted stripes), and 6M treatment (grey, horizontal stripes) groups at 30°C and 35°C. The 40°C trial data are not shown. Error bars represent standard deviation.
Discussion
In this study, the effects of trimethylamine N-oxide on the thermotolerance in Caenorhabditis elegans were investigated, and determined to be both dosage and temperature dependent. At 30°C, 3M TMAO treatments appeared to increase the thermotolerance significantly. On the contrary, 3M TMAO treatments appeared to drastically decrease the stress response in 35°C conditions. Additionally, at 30°C 6M TMAO treatments decreased the thermotolerance significantly, causing the low survival percentage. However, at 35°C 6M TMAO dilutions appeared to increase the thermotolerance slightly. In trial 3, the worms immediately had a 0% survival rate in 40°C conditions. It was determined that 40°C, 20°C above the temperature in which they thrive, was a severely disadvantageous environment for C. elegans. There were two replicate plates in the second trial that showed sudden death of all worms after two hours. Since the other plates at this temperature did not show this drastic change in survival rate, it was determined that an outside factor was responsible for the death of the worms. There were two unsuccessful egg preparations due to a technical error, which resulted in a delay in performing subsequent trials. The protocols for egg preparation were then slightly altered in an attempt to successfully recover eggs. In addition, while preparing eggs for trial one, hypochlorite solution was used for transfer rather than M9 buffer, thereby causing death of the L1 larvae. Based on the results of the experiment, it is a possibility that when the worms were under a constant heat, they resorted to burrowing into the agar in search of a cooler environment. This assumption was made while observing the worms for a survival count. This would explain why the survival rate quickly decreased and then slowly rose back up in first trial at the 6th hour, and the second trial at the 2nd hour. Although certain concentrations of exogenous TMAO increased or decreased the thermotolerance in C. elegans, the results indicate that the presence of elevated levels may also be connected to stress response in other organisms as well. High levels in humans are known to have detrimental effects on health (Wang et al., 2011). However, perhaps the metabolite affects the human body in other ways beyond what has been studied. In future studies, further investigation of the relationship between TMAO concentration and temperature on the survival rate in C. elegans may provide further insight as to the function of endogenous TMAO.
Acknowledgements
We would like to thank Daniel Pinolini and Dr. Bala Subrahmanya Chakravarthy Koritala for their guidance and indispensable assistance with our research project. Additionally, we would like to thank Dr. Amy Savage, Dr. Kwangwon Lee and the members of the Lee Lab for their help and for allowing us to utilize their space.
References
Atherton, H.J., Jones, O.A.H., Malik, S., Miska, E.A., and Griffin, J.L. (2008). A comparative metabolomic study of NHR-49 in Caenorhabditis elegans and PPAR-α in the mouse. FEBS Letters 582, 1661–1666.
Bockus, A.B., and Seibel, B.A. (2016). Trimethylamine oxide accumulation as a function of depth in Hawaiian mid-water fishes. Deep Sea Research Part I: Oceanographic Research Papers 112, 37–44.
Brenner, S. (1974). The Genetics of Caenorhabditis Elegans. Genetics 77, 71–94.
Brunquell, J., Bowers, P., and Westerheide, S.D. (2014). Fluorodeoxyuridine enhances the heat shock response and decreases polyglutamine aggregation in an HSF-1-dependent manner in Caenorhabditis elegans. Mechanisms of Ageing and Development 141–142, 1–4.
Hirani, N., Westenberg, M., Seed, P.T., Petalcorin, M.I.R., and Dolphin, C.T. (2016). C. elegans flavin-containing monooxygenase-4 is essential for osmoregulation in hypotonic stress. Biology Open 5, 668–668.
Hunt, P.R., Son, T.G., Wilson, M.A., Yu, Q.-S., Wood, W.H., Zhang, Y., Becker, K.G., Greig, N.H., Mattson, M.P., Camandola, S., et al. (2011). Extension of Lifespan in C. elegans by Naphthoquinones That Act through Stress Hormesis Mechanisms. PLoS ONE 6, e21922.
Morimoto, R.I. (1998). Regulation of the heat shock transcriptional response: cross talk between a family of heat shock factors, molecular chaperones, and negative regulators. Genes & Development 12, 3788–3796.
Rodriguez, M., Snoek, L.B., De Bono, M., and Kammenga, J.E. (2013). Worms under stress: C. elegans stress response and its relevance to complex human disease and aging. Trends in Genetics 29, 367–374.
Seibel, B.A., and Walsh, P.J. (2002). Trimethylamine oxide accumulation in marine animals: relationship to acylglycerol storagej. Journal of Experimental Biology 205, 297–306.
Van Hecke, T., Jakobsen, L.M.A., Vossen, E., Guéraud, F., De Vos, F., Pierre, F., Bertram, H.C.S., and De Smet, S. (2016). Short-term beef consumption promotes systemic oxidative stress, TMAO formation and inflammation in rats, and dietary fat content modulates these effects. Food Funct. 7, 3760–3771.
Yancey, P.H., Gerringer, M.E., Drazen, J.C., Rowden, A.A., and Jamieson, A. (2014). Marine fish may be biochemically constrained from inhabiting the deepest ocean depths. Proceedings of the National Academy of Sciences 111, 4461–4465.
Zheng, S.-Q., Ding, A.-J., Li, G.-P., Wu, G.-S., and Luo, H.-R. (2013). Drug Absorption Efficiency in Caenorhabditis elegans Delivered by Different Methods. PLoS ONE 8, e56877.
Zhou, K.I., Pincus, Z., and Slack, F.J. (2011). Longevity and stress in Caenorhabditis elegans. Aging (Albany NY) 3, 733–753. Yeung, C.K., Adman, E.T., and Rettie, A.E. (2007). Functional characterization of genetic variants of human FMO3 associated with trimethylaminuria. Archives of Biochemistry and Biophysics 464, 251–259.
Wang, Z., Klipfell, E., Bennett, B.J., Koeth, R., Levison, B.S., DuGar, B., Feldstein, A.E., Britt, E.B., Fu, X., Chung, Y.-M., et al. (2011). Gut flora metabolism of phosphatidylcholine promotes cardiovascular disease. Nature 472, 57–63.
Journal of Biological Sciences at Rutgers Camden (JBS) is licensed under a Creative Commons Attribution-NonCommercial-ShareAlike 4.0 International License