Stephanie Emerson, Humaira Khan, Teresa Osorio, Lisa Traczyk
Department of Biology, Rutgers University, Camden, NJ. 08102
Abstract
Alzheimer’s Disease (AD) is characterized by an accumulation of amyloid-beta (Aβ) plaques between neurons and intracellular neurofibrillary tangles caused by the hyperphosphorylation of tau protein. Since there is no known cure for AD, research into the effects of Aβ plaques and tau tangles could lead to better treatment options and understanding of the mechanisms behind this disease. Researchers are currently studying the effects of sleep deprivation in an AD model using Drosophila melanogaster expressing Aβ, amyloid precursor protein (APP): β-site APP cleaving enzyme-1(BACE), or hyperphosphorylation of tau proteins. However, in a D. melanogaster Tau mutant (TM) AD model, the precise role of sleep deprivation in short term memory retention is currently unknown. In this study we aim to show how disrupted sleep will enhance short term memory perturbations, thus decreasing memory retention in a D. melanogaster TM AD model. We found no significant differences in the average avoidance times for both sleep disturbed and non-sleep disturbed TM groups. Therefore, our results demonstrate that disrupted sleep will not enhance short term perturbations in a D. melanogaster TM AD model. Moreover, our study demonstrates the need to further investigate the pathway responsible for the interactions between sleep and memory in Alzheimer’s Disease.
Introduction
Alzheimer’s Disease (AD) is one of the most common forms of dementia. In 2018, AD was the sixth leading cause of death in the United States, and the fifth leading cause of death in Americans aged 65 or older (Barthélemy et al., 2020). Alzheimer’s Disease is characterized by the accumulation of amyloid-beta (Aβ) plaques between neurons and intracellular neurofibrillary tangles caused by hyperphosphorylation of tau protein (Tue et al., 2020). The accumulation of Aβ plaques between neurons causes a break in neuronal connections leading to neurodegeneration. The neurofibrillary tau tangles block the transport of nutrients, motor proteins, and other essential molecules within the cell (Mietelska-Porowska et al., 2014). As the disease progresses, the accumulation of Aβ plaques and tau tangles results in symptoms such as disrupted sleep, loss of memory, and difficulty completing everyday tasks. Since there is no known cure for AD, research into understanding the effects of Aβ plaques and tau tangles could lead to better treatment options. Researchers are currently studying if improving quality of sleep may serve as a therapeutic method to decrease or slow the progression of memory loss (Dissel et al., 2016). We utilized the model organism, Drosophila melanogaster, to study how sleep disruption affects tau-specific memory dysfunction.
Drosophila melanogaster serves as a model organism for human neurodegenerative brain disorders like AD due to their similarities in anatomical structure to the human brain in aspects such as neuronal connection as well as cellular signaling, synthesis, and death (Tue et al., 2020). Drosophila melanogaster is small and capable of producing a large number of offspring during its short eight-week life cycle (Pulver & Berni, 2012). Additionally, researchers have developed genetic techniques to manipulate gene expression in D. melanogaster (Pulver & Berni, 2012). Given their short life cycle and the advancements in manipulation of gene expression, D. melanogaster is ideal for behavioral experiments because the genes involved with AD can easily be observed (Ali et al., 2011). Additionally, D. melanogaster has a favorable 12:12 hour light: dark sleep cycle for studying sleep disturbances (Dubowy and Sehgal, 2017). Meanwhile, vertebrate species such as mice display more fragmented sleep patterns (Tue et al., 2020). Therefore, D. melanogaster is a suitable model for investigating the effects of sleep deprivation on memory in a Tau mutant (TM) AD model.
Although there is no cure for AD, improving quality of sleep may decrease the development of symptoms related to AD like dementia. Sleep deprivation increases the likelihood of developing dementia in older healthy women suggesting that sleep enhancement may serve as a therapeutic method to decrease or slow the progression of memory loss in patients with AD (Gj et al., 2011). Current research suggests that D. melanogaster AD models such as tau and amyloid precursor protein (APP): β-site APP cleaving enzyme-1(BACE) models modify sleep pathways differently (Dissel et al., 2016). Given that disrupted sleep is a commonly reported symptom in AD patients, studying the effects of disrupted sleep on memory retention could lead to better treatment options and understanding of the disease.
While there have been extensive studies on sleep and the Aβ D. melanogaster model, there are still gaps in understanding how sleep disturbances affect memory retention in a Tau mutant. Recent studies have found that enhancing sleep can reverse memory deficits in flies expressing either Aβ, APP: BACE, or human Tau (Dissel et al., 2016). In contrast, our study aims to examine the effects of sleep disturbances on memory retention in D. melanogaster Tau mutants. We hypothesize that disrupted sleep will decrease short-term memory retention in a D. melanogaster Tau mutant Alzheimer’s Disease model. This hypothesis was tested using a Drosophila ethoscope to deprive D. melanogaster of sleep. Following a night of sleep deprivation, D. melanogaster were tested for memory retention using the Aversive Phototaxic Suppression (APS) assay (Le Bourg & Buecher, 2002). We found no significant differences in the average avoidance time between sleep disturbed and non-sleep disturbed TM groups. This suggests disrupted sleep does not enhance short term memory perturbations in a D. melanogaster TM AD model.
Materials and Methods
Fly lines and maintenance
Oregon-R flies (wildtype) were obtained from Dr. Kwangwon Lee’s lab at Rutgers University-Camden. The stock was cultured and maintained in a cornmeal-based media at room temperature with their 12 hour light: 12 hour dark sleep cycle for an average of 10 days. When larvae were observed, the adult flies were separated so the larvae could grow and mature. After adult flies emerge from pupal cases, they were separated based on gender. One to two week old adult flies were used to conduct this study.
UAS-hTau (BL#51362) and OK371-Gal4 (BL#26160) fly lines were obtained from Bloomington Stock Center. Gal-4 serves as a transcription factor that activates transcription of its target gene by binding to UAS regulatory sites (Pulver & Berni, 2012). In D. melanogaster, when UAS-hTau and OK371-Gal4 lines are crossed, the progeny are expected to express Gal-4 in tau proteins; thus, creating a D. melanogaster Tau mutant to study Alzheimer’s disease (Fernius et al., 2017). Male Gal-4 and female UAS-hTau flies were maintained in a cornmeal-based media at room temperature with their favorable 12 hour light: 12 hour dark sleep cycle for an average of 10 days. When larvae were observed, the adult flies were separated so the larvae could grow and mature. After adult flies emerged from pupal cases, F1generation adult flies were separated based on gender. One to two week old adult flies were used to conduct this study.
Experimental groups
Four groups of flies were observed in this experiment. Two groups served as controlled independent measure groups where the fly lines, wildtype (WT) (n = 8) and Tau mutant (TM) (n = 9), experienced no sleep disturbances during the 12-hour dark cycle. The two experimental groups consisted of the fly lines, WT (n = 8) and TM (n = 9), with targeted sleep disturbances during the 12-hour dark cycle. Following a night of sleep disturbances, individual flies from each group were tested for memory retention with the use of Aversive Phototaxic Suppression Assay. Flies were not separated based on sex for the experimental study as it was determined there were no significant differences between gender relating to avoidance time in previous studies (Arman et al. 2020).
Sleep Disturbances
A Drosophila ethoscope was used as the sleep disturbance apparatus (Fig. 1a). It was assembled as described in the original documentation provided by the Gilestro Laboratory in London (Geissmann et al., 2017). The ethoscope was chosen because it was an affordable apparatus that could be easily 3D printed and utilizes Raspberry Pi microcomputers to deliver behaviorally triggered stimuli to flies in a feedback-loop mode (Geissmann et al., 2017). The ethoscope contains a camera powered by a Raspberry Pi microcomputer placed over an arena containing 20 glass tubes, each containing an individual fly. An infrared led light was reflected in a light box under the flies to provide enough light for the camera to detect the fly’s locomotive activity without disturbing the fly’s night cycle. The data was collected by the ethoscope and sent via Wi-Fi to a computer referred to as the node, which contains software created by the Gilestro Laboratory, to control and monitor the ethoscope.
The sleep disturbance module was implemented as reported (Geissmann et al., 2017). The module consisted of 10 motors placed below the light box and arena holding the flies. A small rubber O-ring was placed over every other tube containing a fly and stretched over a pulley on the motor (Fig. 1a). The experimental groups of flies were in tubes that contained a rubber O-ring connecting them to a motor which allowed them to be disturbed for 12 hours during the night cycle. In contrast, the control groups of flies were placed in tubes not connected to the motors so they could sleep undisturbed. The motors were triggered to turn on by the Raspberry Pi when the camera detected an individual fly immobile for 20 seconds. This allowed the ethoscope to deliver targeted sleep disturbances to individual flies as it was beginning to fall asleep. Time was recorded in Zeitgeber time (ZT) with the light cycle beginning at ZT 0 and the dark cycle beginning at ZT 12.

Figure 1: Experimental Design a) Diagram of assembled Drosophila ethoscope and sleep deprivation module adapted from Geissmann et al. (2017). It was used to track the locomotive activity of the flies and to disturb the sleep of the given groups using rubber O-rings connected to a motor. b) Diagram of T-maze adapted from Arman et al. (2020). The dark chamber was covered with electric tape and the light chamber was illuminated with a light source. The T-maze was used to conduct APS. Figure created with BioRender.com.
Phototaxis test
Drosophila melanogaster naturally moves toward light which means they are positively phototaxic (Kain et al., 2012). This phototactic ability can be studied by using a T-maze (Fig. 1b). The T-maze consisted of a center column with a trap door and two independent chambers, a dark and a light chamber (Fig. 1b) (Ali et al., 2011). To form the chambers, two vials were connected to opposite ends of the middle chamber. The vial on the left served as the dark chamber in which was wrapped with electric tape. The vial on the right served as the light chamber where a light bulb was placed at the end of the chamber. The middle chamber consisted of a 3D printed chamber with a trap door; this prevented flies from entering the light chamber.
To test phototactic behavior, flies from each experimental group were individually placed in the dark chamber with the trap door closed and a fixed red-light source turned on for 30 seconds. After 30 seconds of acclimation, the red-light source was turned off, the white light source was turned on, and the trap door that separates the two chambers was opened. This allowed the fly to access the light chamber. If the fly walks to the lighted chamber within 30 seconds, it is considered positively phototactic (Ali et al., 2011). Only flies that displayed phototactic ability were used in the Aversive Phototaxic Suppression Assay (APS)
Aversive Phototaxic Suppression (APS)
To train flies and analyze the effect of sleep disturbances on short-term memory retention in a D. melanogaster AD model, APS was used. Here, flies learn to associate light, the conditioned stimulus (CS), with an aversive stimulus, the unconditioned stimulus (US) (Le Bourg & Buecher, 2002). In this study, quinine hydrochloride dihydrate was used as an aversive stimulus. This association is known as CS-US which allows one to study learning and memory behaviors in D. melanogaster (Le Bourg & Buecher, 2002). The 0.1 M quinine solution was prepared by diluting 1.98 g of quinine hydrochloride with 50 mL of distilled water (Seugnet, 2009). The T-maze from the phototaxis test (Fig. 1b) was used to conduct this memory assay.
The memory assay was performed at ZT 8 following the procedure conducted for phototaxis ability. However, during acclimation time, 180 µL 0.1 M of quinine solution was pipetted into the lighted chamber on filter paper. After 30 seconds, the trap door was opened, and the white light source was turned on. This allowed the fly to walk into the quinine-coated light chamber. After one minute, the fly was tapped back into the dark chamber, and then the trap door was closed. For each subsequent trial, time was recorded from the start of the trial to the time the fly entered the quinine-coated light chamber. If a fly failed to walk to the light chamber twice after 90 seconds, it was recorded as “Pass” indicating the fly displayed learning ability. These trials served as the training and conditioning phase in which WT flies will associate the light chamber with taste and avoid it; meanwhile, flies with compromised learning capacities will not make this association (Le Bourg & Buecher, 2002). Therefore, this test will help determine the effects of sleep deprivation on short-term memory retention in a D. melanogaster TM AD model.
Results
Sleep deprivation differentially affects locomotive activity in Tau mutant and wildtype flies
To investigate if disrupted sleep decreased short-term memory in a D. melanogaster TM AD model, the activity of all WT and TM flies were first monitored and analyzed using a Drosophila ethoscope before conducting the APS assay (Fig. 2). An ANOVA test was conducted to determine differences in average locomotive activity of WT and TM groups (ANOVA; p < 0.05). A Tukey’s HSD test between WT without sleep disturbances (26.21 +/- 5.89 AU) and TM without sleep disturbances (27.18 +/- 5.42 AU) demonstrated no significant differences (Fig. 2, Tukey’s HSD, p > 0.05). Significant differences were observed between all other group combinations. Specifically, For WT with sleep disturbances (88.21 +/- 6.34 AU) and without sleep disturbances (26.21 +/- 5.89 AU) significant differences were found (Fig. 2, Tukey’s HSD, p < 0.05) indicating that sleep disturbance had an impact on activity. TM groups with sleep disturbances (51.25 +/- 4.78 AU) and without sleep disturbances (27.18 +/- 5.42 AU) were also significantly different (Fig. 2, Tukey’s HSD, p < 0.05), indicating that sleep disturbances in combination with AD result in increased locomotive activity. Lastly, WT flies with sleep disturbances (88.21 +/- 6.34 AU) exhibited significantly higher locomotive activity than TM flies with sleep disturbances (51.25 +/- 4.78 AU) (Fig. 2, Tukey’s HSD, p < 0.05), demonstrating that sleep disturbances affect WT and TM fly lines differently.
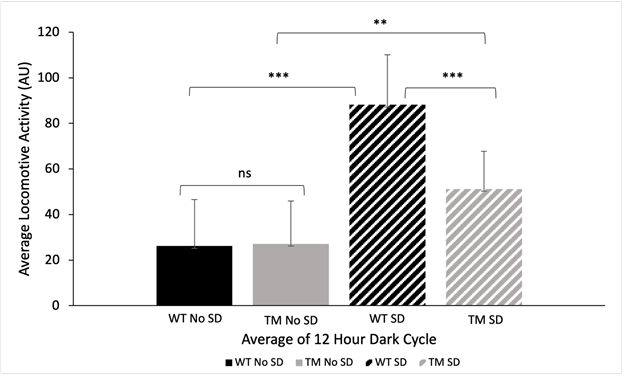
Figure 2: Night Cycle Locomotive Activity. Average locomotive activity of D. melanogaster wildtype and Tau mutant Alzheimer’s Disease model during a 12-hour dark cycle with and without sleep disturbances as measured by the Drosophila ethoscope in arbitrary units (AU). Average time was recorded from 13.5 – 0.5 ZT during the night cycle. An ANOVA test was conducted to determine differences in average locomotive activity of WT and TM groups (ANOVA; p < 0.05). A Tukey’s HSD test was conducted and demonstrated no significant differences between WT without sleep disturbances (26.21 +/- 5.89 AU) and TM without sleep disturbances (27.18 +/- 5.42 AU) (Fig. 2, Tukey’s HSD, p > 0.05). An ANOVA test was conducted to determine differences in average locomotive activity of WT and TM groups (ANOVA; p < 0.05). A Tukey’s HSD test was conducted and demonstrated no significant differences between WT without sleep disturbances (26.21 +/- 5.89 AU) and TM without sleep disturbances (27.18 +/- 5.42 AU) (Fig. 2, Tukey’s HSD, p > 0.05). Significant differences with a p-value less than 0.05 are indicated with a single asterisk, significant differences with a p-value less than 0.01 are indicated with two asterisks, and significant differences with a p-value less than 0.001 are indicated with three asterisks. The use of “ns” indicates a p-value greater than 0.05. Error bars represent standard deviation.
Sleep deprivation differentially affects memory and learning behaviors in Tau mutant and wildtype flies
Following a night in the Drosophila ethoscope, each of the four groups of flies were evaluated for memory and learning ability using the APS assay. The first two trials demonstrate the results for the phototaxis test, trial three exhibits the results when groups were initially exposed to the aversive stimulus, and the four subsequent trials demonstrate the results for APS (Fig. 3a and 3b). In order to measure memory and learning ability, avoidance time was recorded from the start of the trial until the fly crossed over into the light chamber. The average avoidance times for each trial of the four experimental groups can be observed in Figures 3a and 3b.
The WT flies with and without sleep disturbances did not demonstrate significant differences in avoidance time from trials 1-7 (ANOVA; p > 0.05). Trials 4-7 were examined separately to analyze learning and memory following the phototaxic test in trials 1-2, and the first exposure to the aversive stimulus in trial three. Significant differences in avoidance time between WT flies with sleep disturbances (36.89 +/- 4.05 s) and WT flies without sleep disturbances (65.59 +/- 5.72 s) were observed from trials 4-7 (ANOVA; p < 0.05). This indicates that there are significant differences in learning ability between these two groups in these trials. Likewise, TM flies with and without sleep disturbances did not demonstrate significant differences in avoidance time from trials 1-7 (ANOVA; p > 0.05). This trend was also observed in trials 4-7 (ANOVA; p > 0.05), indicating no significant differences in avoidance time between these two groups from trials 4-7 (Fig. 3b).
To further investigate differences in memory and learning behaviors, the avoidance times from trials 4-7 were averaged and shown in Fig. 3c. T-tests were conducted to compare the different experimental groups to reveal information about learning trends. A t-test conducted between WT flies with sleep disturbances (36.89 +/- 4.05 s) and WT flies without sleep disturbances (65.59 +/- 5.72 s) revealed significant differences in avoidance time, indicating sleep disturbance does play a role in memory in WT flies (t-test; p < 0.05). Furthermore, TM flies without sleep disturbances (42.32 +/- 6.69 s) demonstrated significant differences in avoidance time in trials 4-7 from WT flies without sleep disturbances (65.59 +/- 5.72 s), confirming the effect of AD in memory (t-test; p < 0.05). A t-test conducted between WT with sleep disturbances and TM with sleep disturbances showed no significant differences (t-test; p > 0.05). This indicates that sleep disturbance affects learning and memory behaviors in both fly lines similarly. Lastly, a t-test conducted between TM flies with (43.75 +/- 4.05 s) and without sleep disturbances (42.32 +/- 6.69 s) revealed no significant differences in avoidance time (t-test; p > 0.05). This indicates that sleep disturbances did not affect memory in the TM fly line.
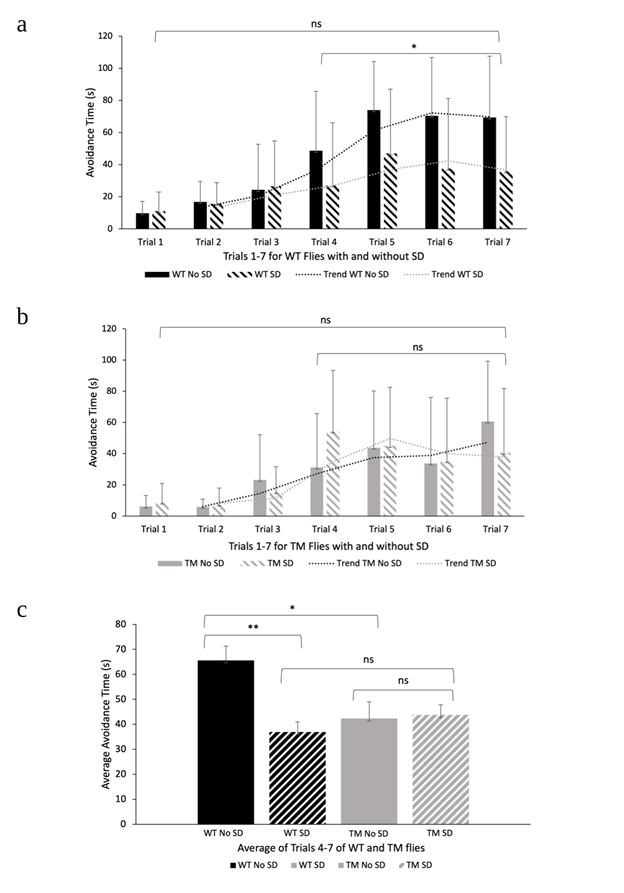
Figure 3: Phototaxis Test and Aversive Phototaxic Suppression Assay. a) Average avoidance times for D. melanogaster WT flies with and without sleep disturbances in APS (n = 8). An ANOVA was conducted to evaluate differences in avoidance time between non sleep disturbed and sleep disturbed WT groups (ANOVA; p > 0.05 for trials 1-7. ANOVA; p < 0.05 for trials 4-7). Error bars represent standard deviation. b) Average avoidance times for D. melanogaster TM with and without sleep disturbances in APS (n = 9). An ANOVA was conducted to evaluate differences in avoidance time between non sleep disturbed and sleep disturbed TM groups (ANOVA; p > 0.05 for trials 1-7 and trials 4-7). Error bars represent standard deviation. c) Average avoidance times for WT and TM D. melanogaster flies with and without sleep disturbances in APS (n = 8; n = 9) in trials 4-7. A t-test was conducted to investigate the effects of sleep disturbance on avoidance time between WT flies with and without sleep disturbances for trials 4-7 (t-test; p < 0.05). This was also done for TM flies with and without sleep disturbances (t-test; p > 0.05). An additional t-test was conducted to examine the effects of AD on avoidance time between the WT and TM non-sleep disturbed groups (t-test; p < 0.05). Finally, a t-test conducted between WT and TM with sleep disturbances did not show any significant differences (t-test; p > 0.05). Significant differences with a p-value less than 0.05 are indicated with a single asterisk, significant differences with a p-value less than 0.01 are indicated with two asterisks, and significant differences with a p-value less than 0.001 are indicated with three asterisks. The use of “ns” indicates a p-value greater than 0.05. Error bars represent SEM.
Discussion
The purpose of this study was to investigate if sleep deprivation will decrease memory retention in a D. melanogaster Tau mutant Alzheimer’s disease model. Our results show that sleep disturbance significantly decreased avoidance time for Oregon-R flies (Fig. 3c). While the avoidance time increased with subsequent trials for sleep disturbed Oregon-R flies (Fig. 3a), it was significantly less than Oregon-R flies without sleep disturbance (Fig. 3c). This observation indicates that sleep disturbance impairs D. melanogaster from suppressing their phototactic ability to associate the conditioned stimulus (CS) with an aversive stimulus. This finding was expected because recent studies have demonstrated that one day of sleep disturbance significantly impairs short term memory in Drosophila (Li et al., 2009). Therefore, we conclude that sleep deprivation significantly decreases memory and learning performance in D. melanogaster.
Additionally, we can conclude that the TM AD model significantly impaired learning and memory in D. melanogaster. TM flies with and without sleep disturbances exhibited significant decreases in learning and memory behaviors in comparison with WT flies with no sleep disturbances (Fig. 3c). In contrast to the WT groups, the data collected from this experiment did not demonstrate significant differences between the two TM groups. These results were not expected because previous studies have concluded that sleep enhancement reverses memory deficits in D. melanogaster Aβ and APP: BACE AD models (Dissel et al., 2016). While sleep disturbances did not contribute to lower avoidance times in TM flies (Fig. 3b, 3c), there is still significance in this observation. Our results suggest there is a certain limit to which learning, and memory is impaired in a D. melanogaster Tau mutant. Our experiment set out to study the impact of sleep disturbances and tau hyperphosphorylation on memory, but the extent to which each of these factors contribute to poor learning ability remains poorly understood. Currently, preliminary research suggests that chronic sleep may impair hippocampus-dependent memory and increase tau accumulation in a mouse model of Alzheimer’s Disease (Rothman et al., 2013) However, whether these two factors play a synergistic role in decreasing memory or if one exhibits dominance over another is unknown. Exploring the limits in which sleep disturbance affects learning and memory behaviors in Alzheimer’s Disease could be an interesting topic for future research.
Although the data collected from APS did not support our hypothesis, we can conclude that the Drosophila ethoscope is a model apparatus to disturb sleep and track locomotive activity during the 12:12 hour dark and light cycle. There were significant differences observed between the two groups, sleep disturbed and non-sleep disturbed, of both WT and TM fly lines (Fig. 2). Additionally, the data collected from the non-sleep disturbed groups is in accordance with previous studies in which D. melanogaster peak activity occurs around dusk and dawn (Dubowy and Sehgal, 2017). In contrast to the non-sleep disturbed groups, the two experimental groups did not display the same significant decrease in activity following lights out (Fig. 2). Our results indicated that there were significant differences between the WT sleep disturbed group and the TM sleep disturbed group suggesting disrupted sleep may differentially affect the locomotive activity of the two fly lines (Fig. 2). This could potentially account for the differences observed in this study. However, further research is needed to fully understand the reasoning behind these differences.
Sleep is essential for cognitive performance and important for a person’s wellbeing (Maquet, 2001). The findings of this study demonstrate that sleep disturbance significantly affects cognitive activities such as memory and learning ability in D. melanogaster to levels evident in TM AD flies with and without sleep disturbance (Fig. 3c). These results are evident in current research which suggests mice exposed to chronic sleep deprivation demonstrated elevated levels of phosphorylated tau protein and impaired cognitive performance (Rothman et al., 2013). Additionally, research has shown patients with insomnia have an increased risk of developing AD while patients with sleep disordered breathing have an increased risk of developing AD and dementia (Shi et al., 2018).
Although our results do not suggest that sleep disturbance significantly affects memory in a D. melanogaster TM AD model, the inconsistencies in avoidance times provide valuable information concerning learning and memory ability. This is evident in Figure 3b where no significant differences in avoidance time for trials 4-7 for TM AD flies with and without sleep disturbances were found. Therefore, we conclude that sleep disturbances do not result in an additional decrease of memory retention in a D. melanogaster TM AD model. However, our findings may have been impacted by small sample sizes. Future research could focus on comparisons between the various D. melanogaster AD models with larger sample sizes. Conducting this study can close the gaps of knowledge between the phosphorylation of tau, amyloid beta, APP: BACE, in relation to sleep and memory.
Acknowledgements
We would like to thank the Rutgers Biology Department for funding our research. We would also like to thank Dr. Joseph Zinski, Dr. Kwangwon Lee and Dr. Nathan Fried for their guidance and feedback in forming and executing our research as well as Ms. Sarah Johnson for ensuring we had the requested materials. Additionally, we would like to extend our thanks to Mr. Cory Trout and the Rutgers Physics Department for the use of the 3D printers and materials. Finally, we would like to thank Mr. Isaac Ramirez for his technical assistance with the setup of the Drosophila ethoscope and Ms. Taqdees Gohar for her assistance with the Aversive Phototaxic Suppression Assay.
References
Arman, H., Gohar, T., Joy, K., Rizvi, Z., and Sohail, R. Omega-3 Fatty Acids in Fish oil Improve Learning and Memory in Drosophila melanogaster. 5.
Barthélemy, N.R., Li, Y., Joseph-Mathurin, N., Gordon, B.A., Hassenstab, J., Benzinger, T.L.S., Buckles, V., Fagan, A.M., Perrin, R.J., Goate, A.M., et al. (2020). A soluble phosphorylated tau signature links tau, amyloid and the evolution of stages of dominantly inherited Alzheimer’s disease. Nat Med 26, 398–407.
Dissel, S., Klose, M., Donlea, J., Cao, L., English, D., Winsky-Sommerer, R., van Swinderen, B., and Shaw, P.J. (2016). Enhanced sleep reverses memory deficits and underlying pathology in drosophila models of Alzheimer’s disease. Neurobiol Sleep Circadian Rhythms 2, 15–26.
Dubowy, C., and Sehgal, A. (2017). Circadian Rhythms and Sleep in Drosophila melanogaster. Genetics 205, 1373–1397.
Fernandez-Funez, P., de Mena, L., and Rincon-Limas, D.E. (2015). Modeling the complex pathology of Alzheimer’s disease in Drosophila. Exp Neurol 274, 58–71.
Fernius, J., Starkenberg, A., Pokrzywa, M., and Thor, S. (2017). Human TTBK1, TTBK2 and MARK1 kinase toxicity in Drosophila melanogaster is exacerbated by co-expression of human Tau. Biology Open 6, 1013–1023.
Geissmann, Q., Rodriguez, L.G., Beckwith, E.J., French, A.S., Jamasb, A.R., and Gilestro, G.F. (2017). Ethoscopes: An open platform for high-throughput ethomics. PLOS Biology 15, e2003026.
Gj, T., (2011). Circadian activity rhythms and risk of incident dementia and mild cognitive impairment in older women. Annals of Neurology, 70(5), 722–732.
Kain, J.S., Stokes, C., and Bivort, B.L. de (2012). Phototactic personality in fruit flies and its suppression by serotonin and white. PNAS 109, 19834–19839.
Le Bourg, E., and Badia, J. (1995). Decline in photopositive tendencies with age in Drosophila melanogaster (Diptera: Drosophilidae). J Insect Behav 8, 835–845.
Le Bourg, E., and Buecher, C. (2002). Learned suppression of photopositive tendencies in Drosophila melanogaster. Anim Learn Behav 30, 330–341.
Li, X., Yu, F., & Guo, A. (2009). Sleep Deprivation Specifically Impairs Short-term Olfactory Memory in Drosophila. Sleep, 32(11), 1417–1424.
Maquet, P. (2001). The role of sleep in learning and memory. Science 294, 1048–1052.
Mietelska-Porowska, A., Wasik, U., Goras, M., Filipek, A., and Niewiadomska, G. (2014). Tau Protein Modifications and Interactions: Their Role in Function and Dysfunction. Int J Mol Sci 15, 4671–4713.
O. Ali, Y., Escala, W., Ruan, K., and Zhai, R.G. (2011). Assaying Locomotor, Learning, and Memory Deficits in Drosophila Models of Neurodegeneration. Journal of Visualized Experiments.
Pulver, S.R., and Berni, J. (2012). The Fundamentals of Flying: Simple and Inexpensive Strategies for Employing Drosophila Genetics in Neuroscience Teaching Laboratories. J Undergrad Neurosci Educ 11, A139–A148.
Seugnet, L., Suzuki, Y., Stidd, R., & Shaw, P. J. (2009). Aversive phototaxic suppression: Evaluation of a short-term memory assay in Drosophila melanogaster. Genes, Brain and Behavior, 8(4), 377–389.
Shi, L., Chen, S.-J., Ma, M.-Y., Bao, Y.-P., Han, Y., Wang, Y.-M., Shi, J., Vitiello, M.V., and Lu, L. (2018). Sleep disturbances increase the risk of dementia: A systematic review and meta-analysis. Sleep Medicine Reviews 40, 4–16.
Rothman, S. M., Herdener, N., Frankola, K. A., Mughal, M. R., & Mattson, M. P. (2013). Chronic mild sleep restriction accentuates contextual memory impairments, and accumulations of cortical Aβ and pTau in a mouse model of Alzheimer’s disease. Brain Research, 1529, 200–208. PubMed.
Tue, N., Dat, T., Ly, L., Duc, V., and Yoshida, H. (2020). Insights from Drosophila melanogaster model of Alzheimer’s disease. Frontiers in Bioscience (Landmark Edition) 25, 134–146.
Journal of Biological Sciences at Rutgers Camden (JBS) is licensed under a Creative Commons Attribution-NonCommercial-ShareAlike 4.0 International License