Conor Rhea, Kyle Norton, Fadima Diawara, Brittany Thompson Department of Biology, Rutgers University, Camden, NJ. 08102
Edited by Joshua Walters
Abstract
This study aims to answer the question of how different diets with different levels of protein affects the physical strength of spider silk. The biological importance of this study is to observe the impact of different diets on the orb weaver spider with hopes to have important implications for engineering and design as well as improving current uses of spider silk such as sutters. Spider silk is considered to be among most durable organic substances on earth due to the toughness and flexibility that it possesses. The Orb-weaver spider, specifically, can produce two different types of silk, called MaSp1 and MaSp2. Which silk is produced is dependent on the type and abundance of amino acids present in the spider’s diet. Orb Weavers produce MaSp1 silk during times of starvation, expressing higher levels of the amino acids alanine and glycine. MaSp2 is produced during times of amino acid abundance, expressing higher levels of glutamine, proline and serine. The aim of this study was to gain insight into this selectivity of amino acid expression by manipulating the spiders’ diets. The spiders’ diets were supplemented with additional amino acids, and the results collected coincide with previous studies which determined that the additional supplementation of the necessary amino acids glutamine, serine, and proline has an effect on the strength of the spider silk produced. Previous studies analyzed the strength of spider silk compositionally through the presence of the amino acids glutamine, proline, and serine, however in this study the physical strength of the silk was tested by determining how much weight each piece of silk could hold. The results determined that amino acid availability in a spider’s diet affects the physical strength of the silk produced. These findings can be used to find the key compositional factors that account for the increase in strength found in this study, which will allow for important implications in engineering and design.
Introduction
Spider silk is considered to be among the most durable organic chemical substance on earth and has attracted human interest for many years. Being able to withstand weathering and chemical degradation, one can imagine the fascination and demand for such material in fields like chemistry, biology, medicine, and even some commercial industries. Aside from toughness and flexibility, spider silk also possesses relatively hypoallergenic qualities. Therefore, spider silk has been very beneficial for its uses in hunting, fishing, and bandages (Römer and Scheibel, 2008). Studying the composition of spider silk can help researchers develop a process for making synthetic silk that is comparable in strength, or even stronger than natural spider silk. In nature, spiders utilize their silk in several different ways, such as, constructing webs, prey capture, egg protection, and as a lifeline to escape from predators (Römer and Scheibel, 2008). At least 130 different structures of spider webs are currently known. Orb weaver spider webs are frequently studied by scientists and contain several different types of silk (Römer and Scheibel, 2008). Araneid or Orb-weaver silk demonstrates immense elasticity and durability. The type of silk made by the orb weaver spider originates in the major ampullate glands and is called MA silk. This particular type of silk consists of two proteins; MA spidroin 1 and 2 (fibroins that are also called MaSp1 and MaSp2). MaSp1 expresses higher levels of the amino acids alanine and glycine, while MaSp2 expresses higher levels of the amino acids glutamine, proline, and serine. Furthermore, the degree to which either MaSp1 and MaSp2 are expressed is dependent on the spiders and the abundance of amino acids in their diet (Blamires et al., 2012). It is likely that with the spiders’ natural diet, MaSp1 and MaSp2 amino acids will be expressed via their silk based on the needs and metabolism of the spider. The method in which Orb-weaver spiders selectively choose the higher expression of MaSp1 or MaSp2 proteins in their silk based on their diet is still poorly understood. It is thought that this selectivity is rooted in how energetically expensive it is for the spiders to synthesize certain amino acids (Blamires et al., 2012). In times of starvation, it has been observed that certain amino acids, alanine and guanine, require lower levels of metabolic energy are synthesized and expressed at higher levels, showing a higher expression of the MaSp1 motif (Blamires et al., 2012). In times of amino acid abundance, the opposite has been observed: higher expression of amino acids glutamine, serine and proline, require more metabolic energy to be synthesized, indicating a shift toward the MaSp2 motif (Blamires et al., 2012). It is this phenomena that our group would like to further examine. In this study, we will investigate the relationship between diet and the strength of silk in orb-weaving spiders. We plan to do this by dividing our population of spiders up into three groups. The first group consisted of spiders being fed a normal diet of Drosophila (two flies per week) with supplemental amino acids being added into their diet. These supplemental amino acids were provided in the form of fibroin, a peptide naturally occurring in spider silk. The second group was a control group, being fed a normal diet of Drosophila and the final group will be a starvation group, being fed a single Drosophila every other week. From here, we plan to test the physical strength of the spider silk from the varying diet groups by anchoring collected silk samples and hanging weight from the anchored silk. We hypothesize that the group of spiders being fed the fibroin supplement will produce stronger silk than the other two groups.
Materials & Methods
Implementation of Diet in Spiders To study the effect of diet on the strength of spider silk, three groups of four Orb Weaver spiders (Nephila) were used, with each individual spider contained in its own habitat. These habitats were comprised of plastic containers with 30 air holes in the lid that were a twentieth of an inch thick to ensure proper ventilation and two cups of potting soil and sticks to supply resources for the spider to anchor its web to. During the course of this experiment, each spider was stored in a temperature-controlled incubator that was programed on a day-night temperature cycle that fluctuates during the course of the day to accurately replicate the temperatures of their natural habitat. The temperature within the incubator was programed to range between 20℃ to 27℃ as well as provide artificial sunlight at 7 a.m. and become dark at 7 p.m. to simulate a normal day-night cycle. This range of temperatures were chosen because it best represents the fluctuation in temperature during the course of the day from sunrise to sunset that the spider would experience living in Florida. The first group of four spiders (treatment group) received a normal diet of two Drosophila flies per week and received cotton balls that were soaked in a solution that was made with 600 mg (3 capsules) of Swanson Ultra NeuroSilk, which is composed of fibroin peptides, dissolved in 200mL of water. This supplement was chosen because of its fibroin composition. Fibroin is composed of glycine, serine, and alanine repeats and makes up the majority of orb weaver MA silk composition. The second group of spiders (control group) received two Drosophila flies per week and a cotton ball soaked in a solution that was made by mixing 300 mg of rice bran and maltodextrin, dissolved into 200 mL of water. The last group (starvation group) received one Drosophila, every two weeks, and received cotton balls that were soaked in the same solution that was given to the control group. The soaked cotton balls in each group were replaced, twice a week, with new soaked cotton balls and placed on squares of aluminum foil; the foil prevented the soil from absorbing the liquid. The cotton balls in the control and starvation groups were soaked in maltodextrin and rice bran solutions because these compounds are the other components of the NeuroSilk supplement. Compensating for liquid Evaporation Measures were taken to account for the amount of fluid evaporation from the artificial environment provided by the incubator. The first measure that was taken was to place beakers of water on each level of the incubator, which created a humidifier-like environment that kept the air moist and prevented excess evaporation from the cotton balls. In addition to the beakers of water, the weight of each cotton ball, soaked in both solutions (NeuroSilk and Maltodextrin/Rice Bran), was measured after soaking each cotton ball in the solutions for 30 seconds to assure that the cotton balls all achieved maximum saturation. The cotton ball that was soaked in the NeuroSilk Solution weighed 13.9 grams, while the cotton ball soaked in the Maltodextrin and rice bran solution weighed 13.2 grams. The two cotton balls were both placed in a container that is identical to the containers housing the spiders, and each container was placed in the incubator. The weight of each cotton ball was taken each day by a group member and documented on a piece of paper to account for the loss in weight due to evaporation. These weights were compared to the weight of the cotton balls being changed out of the spiders’ habitat and it was determined that the weight was very nearly identical, most likely due to that fact that the amount of each solution that each spider drank was not enough to see a visible difference on the scales. Drosophila Preparation Before the spiders were fed the Drosophila, measures were taken so that a steady stock of the Drosophila was kept. This was done by providing an environment in which they can breed and survive. The media for the Drosophila was prepared by taking 10 mL of the Drosophila media and 10 mL of water in two separate graduated cylinders. The media and the water were poured into the same culture vial and the vial was swirled to mix the Drosophila the components. The mixture was given time to settle in which the media absorbed the water and formed a gel. After preparing the media and forming a Drosophila colony, the Drosophila were kept in an incubator at 20℃ to maintain the stock. In order to feed the Drosophila to the spider, the Drosophila were stunned so that they could be easily handled by the experimenter. This was done by chilling an empty 200 mL beaker in the freezer, and once it was sufficiently chilled it is removed from the freezer and was placed on a frozen ice pack. Then the Drosophila were poured from their vial into the empty frozen beaker which stunned the flies. This allowed for the removal of the stunned flies from the beaker and each fly was placed into each spider habitat using a spatula. This process was repeated twice per week for groups 1 and 2, while for group 3, this was done once every two weeks. Silk Collection Ten days was the approximate time needed for the diet changes to start affecting the composition of the spider’s silk (Blamires et al., 2012). Once the ten-day acclimation period had concluded, the silk was collected from each spider habitat using tweezers. This method was adopted after attempts to manually extract the silk from the spiders were unsuccessful due to variation in spider size and the inability to extract the desired length of silk, which was six inches. Every spider spun enough silk themselves to allow for the collection of the proper length of six inches needed to accurately test the strength of the spider silk for each group. Strength Testing The strength of each six-inch silk sample that was collected from the twelve habitats was tested by using a structure created using a 3D printer (Figure 1). The weights for this experiment were made by taking a 6mm x 30 cm pipe cleaner folding it in half and cutting the pipe cleaner into four equal length pieces of 7.5 cm. The weight of the pipe-cleaners was then taken using a scale which was be done by taking the weight of a single pipe cleaner and dividing the weight by 4. Each quarter of pipe cleaner that was cut was bent so that the weights could be placed on the strand of spider silk without them falling off. Paper clips were also used in the testing of the strength of the spider silk in cases that the silk held multiple pipe cleaners and the paperclips took up less space than the pipe cleaners. Like the pipe-cleaners, the paper clips were bent so that they could be easily hung from the silk. In the case of this experiment the recorded weight for the pipe-cleaners was 0.3 g each while the weight for the paperclips was 0.4 g each.
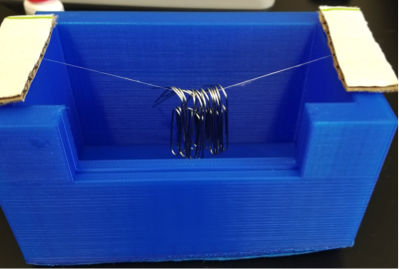
Figure 1. A device measuring the strength of a silk. Six-inch silk sample fixed to 3D printed object for testing. Each six-inch piece of silk was attached to the structure using 3M Ethyl Cyanoacrylate liquid adhesive and strips of cardboard were placed on top of the silk on each end of the structure to ensure that the cause of breaking of the silk was due to the weight placed on the center of silk and not due the adhesive failing.
During the testing a group member held down each piece of cardboard on each end which ensured that the silk remained attached to each end of the structure. During this process a group member recorded the amount of pipe cleaners or paper clips that were hung from each sample of silk before each strand broke due to excessive weight. The strength of silk was determined by taking the sample of silk that was collected from the spiders’ habitat and placing it under a stereomicroscope. The reason for this because some of the samples collected had more than one strand of silk. A picture of the silk was taken using a camera before testing each strand and is looked at using Pixlr Editor to determine the number of strands of silk that are present in each sample of silk. The recorded weight capacity of the silk was divided by the number of strands determined based on the picture taken which determined the amount of weight that each strand of silk could withstand or for a single strand the total weight was recorded (Max weight/ Number of strands of silk). It was determined through personal observations that the spiders were drinking from the cotton balls that were present in each of the spiders’ habitats as the spiders were seen fifteen to twenty times on the cotton balls drinking from them. Results were recorded on a Microsoft Excel Spreadsheet that was saved as a .csv file that was loaded in R Studio. The results were analyzed by first running an ANOVA test followed by a Tukey HSD test to determine if the results of this study were significant or not.
Results
A Shapiro test was performed in RStudio and the data was found to be normally distributed. To test if the different diets influenced the strength of silk produced by the spider, we measured the physical strength of the silk from the spider with different diets (Materials and Methods). We found that NeuroSilk-fed group produced significantly stronger silk than the control group and starvation groups (ANOVA test p < 0.001 and post-hoc TukeyHSD test p < 0.001; Figure 2). The NeuroSilk group was able to hold an average of 0.89 grams more than the average weight capacity of the silk for the Control Group and 1.18 grams more than the average weight capacity for the Starvation Group.
Figure 2. Average weight Capacity of each of the groups tested. Statistical testing was done using ANOVA and post-hoc TukeyHSD in RStudio. Asterisks indicate statistically significant difference when NeuroSilk group is compared to Control and Starvation groups. (***p < 0.001). The silk produced by the NeuroSilk group supported an average of 64% more weight than the control group and an average of 86% more weight than the starvation group.
Discussion
The purpose of this study was to determine whether different diets with varying levels of protein affected the physical strength of spider silk. After testing the physical strength of the silk in either the NeuroSilk, Control, or Starvation groups, the collected results suggest that spiders in the NeuroSilk group had significantly stronger silk (Figure 2). Craig et al establish that MaSp2 silk may contain higher level of amino acid such as serine, glutamine, proline, and alanine which may be the composition of the NeuroSilk group. Meanwhile, the upregulated expression of MaSp1, perhaps was the result of silk produced by the starvation group, which may have contained an increase in glycine and alanine, and a decrease in the serine, glutamine, and proline. Also, the spiders fed with higher protein diets should have stronger silk when compared to the starvation group, as well as less silk production (Craig et al., 2000). The result of this experiment suggests that silk production and silk strength may have something to do with the variation of protein intake. Visual analysis of the silk from each group also shows a clear difference based on the diet that each group of spiders was fed. The appearance of the silk for the NeuroSilk was more visible, having a white tint to the silk. The silk produced for this group was also more abundant than the other groups tested, and silk extraction was easier because the strands of silk stayed intact when removed from the habitat. The silk of the Control group was harder to collect and was less abundant than the silk of the spiders in the NeuroSilk group. The collected silk was semi-sturdy but broke much easier than the silk of NeuroSilk. Lastly, the silk of the Starvation group was the hardest to collect and required delicate extraction due to the fact that the silk broke very easily. The silk produced was less abundant in this group when compared to the NeuroSilk group and it was less visible. Overall, the appearance and durability of the silk in each group, during extraction, suggested a difference in weight capacity before testing. Initially, it was a concern that the variation in spider size could have an influence on the results of the strength testing. To control for this, the variation in spider size was distributed evenly throughout all three groups, effectively abrogating these concerns. These results confirmed our hypothesis that the addition of the NeuroSilk supplement in the spider’s diet would increase strength, while the starvation of the spiders would cause a decrease in physical strength. It seems that the silk produced by the starvation group broke easily when weight was attached to it could be caused by the lower levels of these amino acids like serine, proline, and glutamine when compared to the NeuroSilk group. The results collected in this experiment can be useful in answering the question of how dietary factors affect the physical strength of spider silk. However, further research still needs to be done to find the possible cause as to why the NeuroSilk group of spiders produced stronger silk than the other two groups. Future research that offers a better understanding of the exact mechanism by which spiders synthesize stronger silk and finding the key compositional factors that account for this increase in strength, will allow for important implications in engineering and design. Examples of this could be something such as a microscope superlens. The use of a superlens, constructed out of spider silk, could possibly go past the conventional diffraction limit posed by traditional microscope lenses, making previously ‘invisible’ objects come into view.
Acknowledgements
The authors would like to thank Dr. Kwangwon Lee for giving us this opportunity to perform our own research, Daniel Pinolini for assisting us along the way, and the Rutgers Camden Biology Department for providing a new lab for undergraduate students to work in.
References
Blamires, S.J., Wu, C.-L., and Tso, I.-M. (2012). Variation in Protein Intake Induces Variation in Spider Silk Expression. Page ONE 7, 1-9. Gordon Ramel The Earthlife Web – Spider Life Cycles and Ecology.
Hernández Cruz, D., Rousseau, M.-E., West, M.M., Pézolet, M., and Hitchcock, A.P. (2006). Quantitative Mapping of the Orientation of Fibroin β-Sheets in B. mori Cocoon Fibers by Scanning Transmission X-ray Microscopy. Biomacromolecules 7, 836–843.
Normandeau, J., Van Kessel, C., Nicholson, D., Rahusaar Routledge, B., Fawcett, A., Lim-Cole, L., Condy, C., Sylvain, N., Walker, T., and Borondics, F. (2014). Spider silk protein structure analysis by FTIR and STXM spectromicroscopy techniques. Can. Young Sci. J. 2014, 35–42.
Sashina, E.S., Bochek, A.M., Novoselov, N.P., and Kirichenko, D.A. (2006). Structure and solubility of natural silk fibroin. Russ. J. Appl. Chem. 79, 869–876. Römer, L., and Scheibel, T. (2008). The elaborate structure of spider silk. Prion 2, 154–161.
Journal of Biological Sciences at Rutgers Camden (JBS) is licensed under a Creative Commons Attribution-NonCommercial-ShareAlike 4.0 International License