Donaira Dent, Sashoya Dougan, Chelsea Guarrera, Swetha Samuel
Department of Biology, Rutgers University, Camden NJ 08102
Abstract
Anxiety is the body’s response to any type of change that may cause an emotional, physical, or psychological strain. Although it is a very natural human feeling, an abnormal or unhealthy amount of anxiety may disrupt bodily functions in a negative way. Neurodegenerative diseases are a crucial problem for many worldwide. These diseases are caused by the decomposition of brain structures causing debilitation in those suffering from it. Amyotrophic lateral sclerosis (ALS) is a neurodegenerative disorder which causes extreme deficits in motor dysfunction and can eventually lead to death. There is no known cause as to why individuals develop ALS, other than genetic mutations. However, scientists are continually researching which factors could influence or worsen ALS symptomatology. Although psychological stress, such as anxiety, has been proposed as a risk factor for neurodegenerative diseases such as Mild Cognitive Impairment, Dementia, and Alzheimer’s Disease, studies on the effects of stress on ALS are limited in scope. To address this knowledge gap, we tested whether ALS symptomatology will worsen when introduced to psychologically stressful scenarios among the wild type and four mutant ALS fly lines of Drosophila melanogaster. We used a previously validated approach to induce maximal stress in Drosophila by shaking them for 5-8 hours. We then used the negative geotaxis assay, a behavioral proxy for motor-related deficits in ALS animals. Our results showed that the two mutant strains Superoxide Dismutase, or SOD1 and Fused in Sarcoma, or FUS, had the greatest adversary effect on the existing ALS symptomatology compared to the wild type. Our study also suggests that the knockdown FUS1 gene that is responsible for repairing errors in DNA, showed a significant difference when compared to the knockout SOD1 gene that overexpresses and causes toxicity and protein dysfunction. We believe this experimental design has the potential to test the effects of different stress levels on ALS symptomatology in D. melanogaster which can be used on a larger scale.
Introduction
Each year, around 5,000 people in the United States are diagnosed with the rare disease known as Amyotrophic lateral sclerosis, or ALS (Arthur et al., 2016). ALS is a late-onset fatal neurodegenerative disease that targets motor neurons. As the motor neurons begin to shut down, the brain loses its ability to control muscle movement. This leads to involuntary muscle spasms, loss of speech, and the ability to breathe ultimately causing death. On average, patients will only live 2-5 years after diagnosis (Chio et al., 2009). To date, there is no cure for ALS, but several drugs like Edaravone have been linked to the slowing of the disease and longer life expectancy (Khairoalsindi and Abuzinadah, 2018). The biopsychosocial aspect of human life proposes that psychological and environmental variables can influence neurological disorders on a genetic basis, (Olden et al., 2011). One variable of interest is stress. Stress has been linked as one of the main triggers for seizures in epileptics as it over-sensitizes the inflammatory responses in inflammatory pathways (Espinosa-Garcia et al., 2021) and increases chances of childhood respiratory disorders like asthma (Rosa et al., 2018). Yet not much is known about the connections of stressors on ALS symptomatology (Parkin Kullmann et al., 2018)
Drosophila melanogaster, commonly known as fruit flies, were the model organisms for our experiment. They are versatile animals that can be genetically modified in numerous ways and are quite like humans. In fact, many known human disease-causing genes have a similar match in drosophila homolog (Jennings, 2011). Genetically modified D. melanogaster models have been utilized in the field of human neurodegeneration for three decades, allowing for a rich reference database to back much of our research (Hirth et al., 2010). In addition to the low maintenance cost of sustaining them, D. melanogaster has fast progeny development allowing for multiple trials to be performed without time constraints. D. melanogaster has been beneficial for further understanding the interaction of stress on ALS symptomatology. To study this interaction, we analyzed two common gene mutations known to cause ALS in humans.
Several genes have been studied in correlation to ALS progression. Two genes associated with it are Superoxide Dismutase, or SOD1 and Fused in Sarcoma, or FUS. SOD1 is the most common genetic mutation in individuals with ALS. In normal conditions SOD1 is a gene used to make the enzyme Superoxide Dismutase, they bind to copper and zinc to prevent formation of charged superoxide radicals (Bunton-Stasyshyn et al., 2015). When overexpressed it causes toxicity and protein dysfunction leading to ALS symptomology like muscle weakness (Kunst et al., 1997). FUS is responsible for transcription, it cuts and rearranges mRNA for the production of proteins in the cell. FUS is also responsible for repairing errors in DNA, to avoid genetic damage. FUS mutation results in motor neuron degeneration (Nakaya and Maragkakis, 2018). This gene has also been closely linked to familial derived cases of ALS more than sporadic (Kamelgarn et al., 2018). Consequently, we obtained two mutant D. Melanogaster that explore modifications in SOD1 and FUS through over-expression of the genes.
In this study we sought to investigate the effects of anxiety on ALS symptomology in four different strains of mutant ALS D. melanogaster. We hypothesized that ALS symptomatology will worsen due to heightened exposure to stressful scenarios. To test this hypothesis, ALS symptoms of the wild type and mutants D. melanogaster were characterized under anxiety. Our data suggest that ALS does worsen when under the effects of induced anxiety. SOD1 and FUS1 D. melanogaster strains had the greatest adversary effect on the existing ALS symptomatology under the tested conditions.
Materials & Methods
Wild Type and Mutant Flies
Ten Mackay flies were obtained from Dr. Lee’s laboratory at Rutgers-Camden to help us begin our study. Females lay multiple larvae a day inside the food source. As the larva grew and overcrowding started to occur in the tube, the adult D. melanogaster were transferred into a new tube, this process was repeated again for future progeny. Four fly lines of the SOD1 and FUS genes were obtained from the Bloomington Drosophila Stock Center with the help of Dr. Fried. The rs2 Sod1n64 pp/TM3, Sod1F Sb1 Ser1 (SOD1) includes the Sod1[F] and Sod1[n64] mutations. Each mutation is a single point mutation which manipulates the normal SOD1 protein in D. melanogaster to form more aggregates and decline in enzymatic activity (FB2022_01). SOD1[X-39] e[1]/TM3, Sb[1] Ser[1] (SOD2) contains the SOD[x-39] deletion. It completely knocks out SOD1 so it’s a loss of mutant function (FB2022_01) . Caz[8b]/FM7c, sn[+] (FUS1) is a mutant fly line that contains modifications to CAZ, the fly homolog of the FUS gene. This particular genotype contains a mutation in CAZ that prevents it from being functional . y[1] cv[1] v[1] caz[5g11] f[1]/FM7c (FUS2) animals are similar to the previous strain (FUS1). They, too, produce a mutant caz gene with a mutation that makes it nonfunctional (FB2022_01). We followed the Bloomington Stock Center’s recommendations for fly care after receiving them. In brief, all strains of flies were reared in vials containing cornmeal media which was made using a 1:1 ratio of drosophila media mix and water. The food media was placed in a clean vial ⅔ its length, and the adult flies were placed in and sealed using cotton tissue to allow airflow but prevent escape.
Table 1. Mutant flies used in the current study
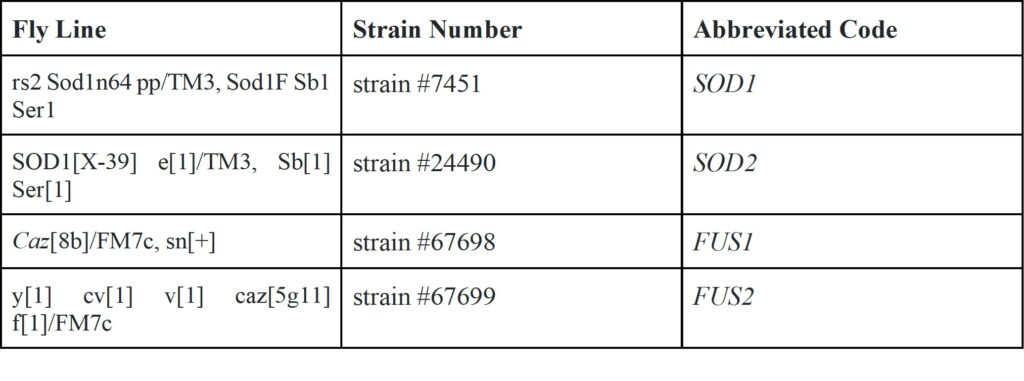
Anxiety Induction
A range of 2 to 10 flies, depending on survival numbers, were placed into clean vials with a wet cotton ball at the bottom and another at the rim of the vial to prevent escape. This was done for each control and stressed group. There were a total of 10 vials each trial. There were 2 vials for each strain. One to be stressed and one that was not stressed, but placed on the hood of the shaker to simulate all the same conditions other than being directly stressed (each set of 2 vials had the same number of flies as their similarly strained counterpart). Each of these tubes, whether they were a control (taped on the hood) or placed on the shaker, were left in the shaker for 8 hours during the first trial, 5 hours during the second trial and 6.5 hours during the third trial (Lall et al., 2019). Once these time intervals would end, the flies were taken out of the shaker and transferred into designated food vials to rest overnight. The negative geotaxis assay was performed with the flies the day after to determine any significant differences in motor function.
Negative Geotaxis Assay
A negative geotaxis assay was used to examine the climbing ability of the control and experimental flies. Although flies can be stressed in other ways such as immobility, heat, and electric shock, this test was used because of its ability to cause neurological changes in behavior and anatomy. Running this assay helped us recognize any decline in the motor function of these flies within the wild type and the four ALS strained flies. To test if the factor of anxiety caused any changes in the motor function of adult Mackay wild-type and mutant flies, we transferred a range of 2 to 10 untested D. melanogaster from their feeding vials to new vials that did not contain food. Using a sharpie, a horizontal line was made 5cm up the tube from the bottom of the vial. Once all the flies to be tested were transferred into the vial, a cotton ball was placed in the rim of the vial to prevent escape, the bottom of the vial is knocked on the table and a timer is started and stopped after the 18 second interval is complete. After this period, the flies that were able to climb and the ones that did not, were recorded. Anxiety induced groups were discarded after three trials were complete. There was a total of three trials (n = 3). For each trial, the negative geotaxis was completed 3 times for each fly group. Data was recorded using an iPhone camera to simplify and increase accuracy during the counting process. A 2-way Anova was run using GraphPad Prism version 8.0.0 for Windows, to find the p-value of the data recorded and a detailed analysis was performed.
Experimental Design
The experiment conducted involved ten groups containing a control and stress group of: Mackay wild type, SOD1 (Sod1[F] and Sod1[n64] mutations), SOD2 (SOD[x-39] deletion), FUS1 (Caz[8b]/FM7c – caz gene mutation) and FUS2 (y[1] cv[1] v[1] caz[5g11] f[1]/FM7c – caz gene mutation). The control groups were given water in new experimental vials and taped horizontally to the hood of the shaker whereas the stress group was given water and then placed in the shaker for 8 and 5 hours respectively without any breaks in between (Lall et al., 2019). The ten groups were then transferred to vials containing food and were left to rest overnight to be able to perform the negative geotaxis assay the following day. To perform the negative geotaxis assay, the groups were transferred into their testing vials in which lines were drawn at the 5cm mark to differ and collect data. Then, the flies were brought all the way down to the bottom by tapping the vial on the table a few times. An 18 second timer was set and during this time, the number of flies that climbed above the mark and the ones that did not, were recorded. The flies that were able to climb above the mark implied that their motor function did not change after the shaking duration. However, the flies that were not able to climb above the mark showed that stress did worsen their motor function. This experiment was repeated for a total of three trials and the data was averaged out for later analysis using a 2-way Anova. Using this data, we were able to determine whether ALS symptomatology would worsen in D. melanogaster, when exposed to the factor of stress.
Figure 1. Illustration of Experimental Design. An overview of the experimental design where five control fly vials were placed against the hood of the shaker during the time that the five experimental fly vials were placed in the shaker. Figure illustration created in BioRender.
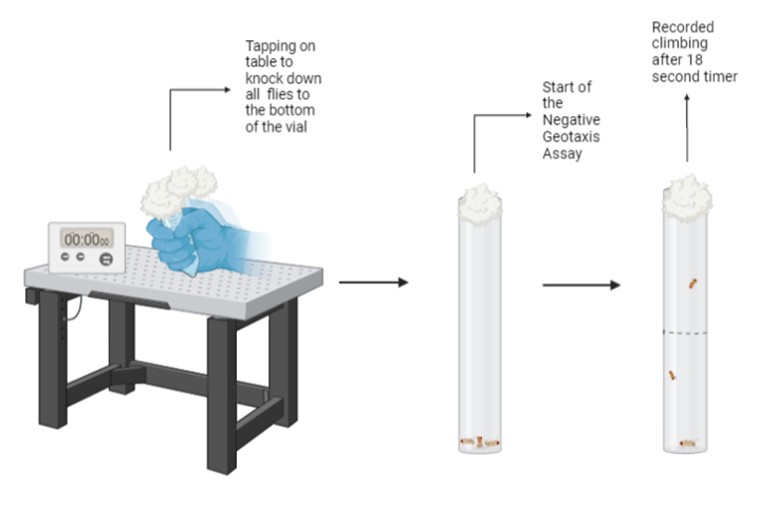
Figure 2. Illustration of Experimental Design. An overview of the Negative Geotaxis Assay where fly vials were tapped on a table to knock the flies down to the bottom of the vial, signifying the start of the 18 second timer. After, the number of flies that climbed past the 5cm mark were recorded for further analysis. Figure illustration created in BioRender
Results
To test whether anxiety worsens ALS symptomatology, a negative geotaxis assay was performed. Anxiety-treated groups of mutants SOD1, SOD2 and FUS1 (refer to experimental design) had decreased motor function compared to the control groups not treated with stress (Figure 3). FUS2 was the only mutant that showed a similar percentage of climbing success compared to the wild type Mackay. Mutant strain SOD1 died in the process. Strains SOD2 and FUS1 showed the lowest climbing ability. Lastly, the only group to show a significant decrease in motor function was FUS1.
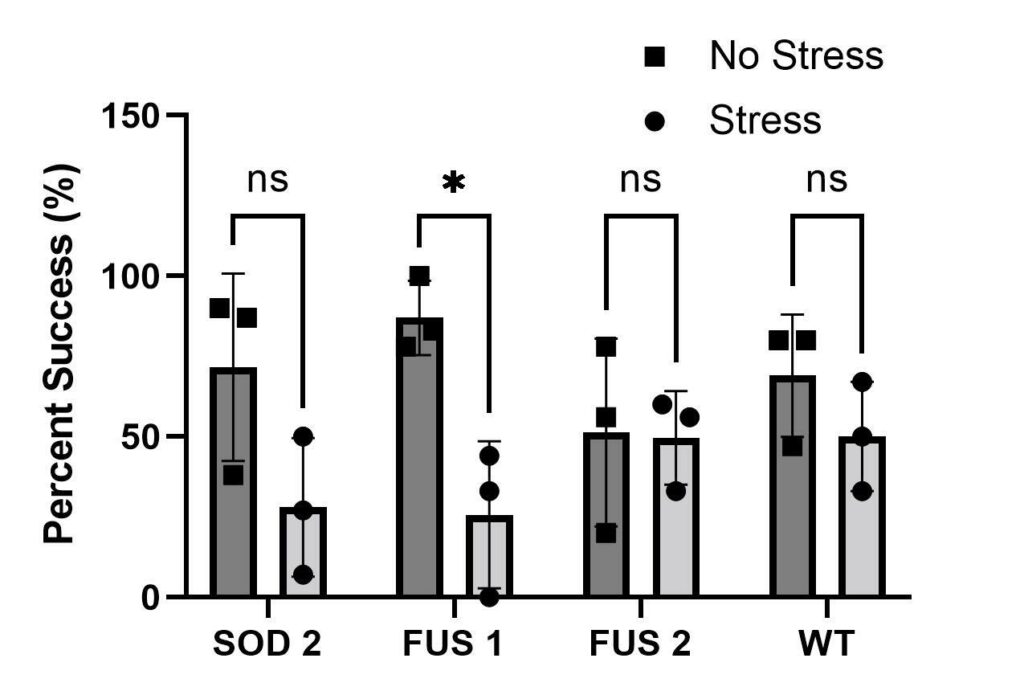
Figure 3. Negative Geotaxis Assay. Bar graph showing overall results of all trials of negative geotaxis assays at 5cm marker (n = 3). D. melanogaster genotypes represented on the X-axis. The percent success of negative geotaxis assay is represented on Y-axis. All strains were located and obtained from the Bloomington Drosophila Stock Center (BCc). We tested two SOD1 genotypes so we labeled them SOD1 and SOD2 for organizational purposes in our experiment. Due to the death of the stress group SOD1, it was removed from the graph. Two FUS genotypes were tested, so they were named FUS1 and FUS2. ALS mutant genotypes represented as SOD1 (BCc#7451), SOD2 (BCc#24490), FUS1 (BCc #67698) & FUS2 (BCc#67699). Our control/Wild-type tested is the Mackay wild type (BCc#25174) shown as WT in the graph.
Discussion
Our study illustrates that the factor of stress does worsen ALS symptomatology in certain ALS mutated D. melanogaster. Within the different strains, SOD1 had the most catastrophic effects when induced with anxiety, resulting in fly death and elimination from our analysis. These deaths may have been due to the strain experiencing a more aggressive type of ALS. The SOD1 gene causes protein dysfunction and muscle weakness that has been linked to be a causative gene of ALS (Valmanis and Rouleau, 2008), and it can be speculated that under elevated anxiety is more easily affected. Despite SOD1 not being analyzed, our hypothesis is supported by the significant decrease in motor function of the FUS1 strain when induced with anxiety. Although there was a significant decrease in motor function of this strain, our study is limited to only understanding organismal stress and not cellular. It is exemplified that ALS symptomology increased under these conditions but not known at the cellular level what caused this increase. The differences in how these mutations affect the organisms, give variability to our results but further research should be done to fully understand what at the cellular level changed under increased anxiety.
To recap, we tested two different SOD1 mutations in D. melanogaster that manipulate (BCc#7451) or completely knockout the level superoxide radicals (BCc#24490) due to the complete knockout of the SOD1 gene. Likewise, testing two different human FUS mutations in D. melanogaster also known as Caz; both FUS1 and FUS2 have mutations in the CAZ gene therefore preventing errors in DNA to be fixed and causing motor neuron degeneration. This study exemplified realistic results that certain ALS genotypes based on severity, can play a role in how anxiety can worsen ALS symptomatology. In addition, the reason for only one strain showing significance, could be that in the second trial the mutant FUS2 and the wild type Mackay showed more success in their negative geotaxis assay in the stress groups than compared to their non-stress groups. This result is contradictory to our hypothesis but occurred due to the paths that the flies were taking in the test tube. Non-stress groups showed more interest in exploring their tube instead of showing typical upward crawling behavior to the cotton ball. Movements consisted of going side to side in the tube or crawling up and down. The reason for this behavior might be due to mixed populations including both male and females. A previous study reported that stress induced female D. melanogaster lost interest in exploring their habitats (Lall et al., 2019). This could support why there was more success of the negative geotaxis assay of FUS2 and wild type (BDSC #25174) when induced with stress.
In addition, we understand that our study had limitations of time and resources. Constant shaking for 4 straight hours instead of 15-minute intervals for longer periods of time could play a role in killing some of the strains. In addition, these results would be more feasible if the mutant strains descended from the same parental line of the wild type strains. Mutants FUS and SOD1 come from a different lineage compared to the Mackay wild type flies, making it more difficult to conclusively compare the results between the ALS mutants and control group. Parental likeness would strengthen the conclusion that the worsening symptoms after stress were indeed caused by the ALS genes and not differing genes between the wild type and mutant groups. The reasons for our decisions revolve around differing schedules amongst group members, lab time and the constraint of conducting the experiment over one semester. There were some issues troubleshooting our experiment in terms of the number of hours spent on shaking, observing mixed populations, and rearing difficulties. To improve this study, more time would be needed to find the most optimal way to induce anxiety, observing male and female flies separately, a larger number of flies tested, and trials conducted to give our study more accurate results by decreasing the chance for randomized results in statistical tests. We hope future studies can be done to further understand why under increased anxiety SOD1 strains resulted in death and others did not and why certain strains of FUS and SOD1 showed significant results and others did not.
The research done in this paper will be beneficial for future studies that take place in more complex organisms, like rodents and may be relevant for research in humans to find out whether stress plays a role in the worsening of ALS symptomatology. We hope in the future, further studies can be done to learn ways to reduce anxiety so those diagnosed with ALS can live a longer and more active lifestyle.
References
Kullmann, J.A.P., Hayes, S., and Pamphlett, R. (2018). Is psychological stress a predisposing factor for amyotrophic lateral sclerosis (ALS)? An online international case-control study of premorbid life events, occupational stress, resilience and anxiety. PLOS ONE 13, e0204424.
Rapid iterative negative geotaxis (RING): a new method for assessing age-related locomotor decline in Drosophila – ScienceDirect.
Chio, A., Logroscino, G., Hardiman, O., Swingler, R., Mitchell, D., Beghi, E., and Traynor, B.G. (2009). Prognostic factors in ALS: A critical review. Amyotroph Lateral Scler 10, 310–323.
Espinosa-Garcia, C., Zeleke, H., and Rojas, A. (2021). Impact of Stress on Epilepsy: Focus on Neuroinflammation—A Mini Review. Int J Mol Sci 22, 4061.
Heller, L. ALS, Amyotrophic Lateral Sclerosis, Lou Gehrig’s disease.
Jennings, B.H. (2011). Drosophila – a versatile model in biology & medicine. Materials Today 14, 190–195.
Khairoalsindi, O.A., and Abuzinadah, A.R. (2018). Maximizing the Survival of Amyotrophic Lateral Sclerosis Patients: Current Perspectives. Neurol Res Int 2018, 6534150.
Kunst, C.B., Mezey, E., Brownstein, M.J., and Patterson, D. (1997). Mutations in SOD1 associated with amyotrophic lateral sclerosis cause novel protein interactions. Nat Genet 15, 91–94.
Lall, S., Mudunuri, A., Santhosh, S., Malwade, A., Thadi, A., K., G., and Dey, S. (2019). Adult crowding induces sexual dimorphism in chronic stress-response in Drosophila melanogaster.
Nakaya, T., and Maragkakis, M. (2018). Amyotrophic Lateral Sclerosis associated FUS mutation shortens mitochondria and induces neurotoxicity. Sci Rep 8, 15575.
Rosa, M.J., Lee, A.G., and Wright, R.J. (2018). Evidence establishing a link between prenatal and early-life stress and asthma development. Curr Opin Allergy Clin Immunol 18, 148–158.
Tolwinski, N.S. (2017). Introduction: Drosophila—A Model System for Developmental Biology. J Dev Biol 5, 9.(2015). An introduction to fruit flies.
Peña-Bautista, C., Casas-Fernández, E., Vento, M., Baquero, M., and Cháfer-Pericás, C. (2020). Stress and neurodegeneration. Clin Chim Acta 503, 163–168.
Bunton-Stasyshyn, R.K.A., Saccon, R.A., Fratta, P., and Fisher, E.M.C. (2015). SOD1 Function and Its Implications for Amyotrophic Lateral Sclerosis Pathology: New and Renascent Themes. Neuroscientist 21, 519–529.
Efimova, A.D., Ovchinnikov, R.K., Roman, A.Yu., Maltsev, A.V., Grigoriev, V.V., Kovrazhkina, E.A., and Skvortsova, V.I. (2017). The FUS protein: Physiological functions and a role in amyotrophic lateral sclerosis. Mol Biol 51, 341–351.
Parkin Kullmann, J.A., Hayes, S., and Pamphlett, R. (2018). Is psychological stress a predisposing factor for amyotrophic lateral sclerosis (ALS)? An online international case-control study of premorbid life events, occupational stress, resilience and anxiety. PLoS One 13, e0204424.
Hirth, F. (2010). Drosophila melanogaster in the Study of Human Neurodegeneration. CNS Neurol Disord Drug Targets 9, 504–523.
Olden, K., Freudenberg, N., Dowd, J.B., and Shields, A.E. (2011). Discovering how environmental exposures alter genes and could lead to new treatments for chronic illnesses. Health Aff (Millwood) 30, 10.1377/hlthaff.2011.0078.
Larkin A, Marygold SJ, Antonazzo G, Attrill H, dos Santos G, Garapati PV, Goodman JL, Gramates LS, Millburn G, Strelets VB, Tabone CJ, Thurmond J and the FlyBase Consortium (2021)
Valdmanis, P. N., & Rouleau, G. A. (2008). Genetics of familial amyotrophic lateral sclerosis. Neurology, 70(2), 144–152.
Kamelgarn, M., Chen, J., Kuang, L., Jin, H., Kasarskis, E.J., and Zhu, H. (2018). ALS mutations of FUS suppress protein translation and disrupt the regulation of nonsense-mediated decay. Proceedings of the National Academy of Sciences 115, E11904–E11913.
Journal of Biological Sciences at Rutgers Camden (JBS) is licensed under a Creative Commons Attribution-NonCommercial-ShareAlike 4.0 International License