Syeda Aiman Nadeem, Maximilian Blank,
Alexa Wilson, Victoria Giarratano, Sandy Lam
Department of Biology, Rutgers University, Camden NJ 08102
Abstract
Epilepsy is a disease caused by an excitation/inhibition imbalance in the brain that leads to hyper synchronous network activity and can result in seizures; such diseases affect millions of people worldwide. While some clinical evidence suggests that chronic pain conditions, such as fibromyalgia, are correlated to the diagnosis of seizures, the potential underlying mechanism that causes those seizures is unknown. Here, we use a specific nerve injury that induces chronic neuropathic pain and measure the effects that it has on the neuronal balance of the brain. The model organism, Drosophila melanogaster has been the subject of various publications regarding seizures and the effects of neuropathic pain, establishing it is a useful model organism. We hypothesized that a bang-sensitive D. melanogaster mutant, julius seizure (jus), would experience an increased susceptibility to seizure levels following a leg amputation known to cause chronic neuropathic pain and a decrease in gamma-aminobutyric acid (GABA) producing neurons. We found that the amputation significantly increased seizure duration from 37.90 s ± 2.20 to 64.42 s ± 11.32 in the jus mutant. Wild type (w1118) and painless (pain) control flies did not experience an increase in seizure duration following injury with baseline levels at 5.87 s ± 0.82 and 1.60 s ± 0.77 respectively. The data suggests that a leg amputation may increase seizure activity in seizure prone patients while also validating D. melanogaster as an animal model to study the mechanisms behind the seizure-pain relationship.
Introduction
Millions worldwide suffer from epilepsy, a disease that is characterized by hyper-synchronous network activity in the brain, typically caused by an excitation-inhibition imbalance. Symptoms from epilepsy, like seizures, can vary greatly. One patient with epilepsy could experience one to two seizures per day, while others can experience hundreds in the same time period. Epilepsy can be associated with mood changes, cognitive impairments, loss of consciousness, and even death. Although some epileptic patients can control their symptoms through the use of anti-epileptic drugs (AEDs) and other treatments, there are many who suffer from treatment-resistant, intractable, epilepsy (NIH, 2022). Approximately 15 million people worldwide are unable to adequately control their seizures with currently available medications (Parker et al., 2012). Understanding the variables that can impact seizure severity is thus imperative for improving patient outcomes.
Chronic pain, like epilepsy, causes distress to millions around the world. Some examples of chronic pain conditions include migraines, arthritis, cancer, and back pain. Recently, researchers have discovered that those with a preexisting chronic pain condition, such as fibromyalgia, are prone to seizures (Tatum et al., 2016). This disease causes a disruption in the brain’s natural chemistry, and thus, seizures can follow (Lee et al., 2018). The connection between chronic pain and its effect on seizure susceptibility is still a novel clinical research topic. By better understanding neuropathic pain and its role in seizure susceptibility, patient suffering can be reduced.
To test this, we designed an experiment to assess the impact of a chronic pain injury on seizure activity of D. melanogaster. This model organism has genes that share similarities with humans and other mammals, allowing their use in the study of human disease mechanisms (He et al., 2022). Furthermore, seizure-like neuronal activity and behaviors, as well as chronic pain is well studied in D. melanogaster (Parker et al., 2012).
We utilized a bang-sensitive (i.e., paralyzes or seizes upon mechanical shock) jus mutant which expresses an increased susceptibility to seizures following high threshold mechanical stimulation due to PBac transgenic insertion in the jus gene (Horne et al., 2017). The jus gene normally results in the production of a two- transmembrane protein that is known to prevent epilepsy. The gene is located at polytene bands 98F10– 99A1 and has been previously identified in the literature as slamdanceiso7.8 (sda). Its exact molecular function is unknown, however, it is known to be involved in mechanosensory behavior and regulation of membrane potential (Fly Base, 2023). The PBac insertion of transposon disrupts the gene and causes loss of function. It has also been discovered that RNAi knockout of this protein can cause seizure-like symptoms after mechanostimulation, partially through a reduction in GABAergic neuronal functioning (Horne et al., 2017). This mutation along with the induction of chronic pain via leg amputation is known to reduce GABAergic neuronal populations, which we hypothesized would increase the GABA-specific effect of the jus mutant.
To represent the negative control group, we used mutated painless flies that have a PBac transgenic insertion in the pain (Dmel\P{EP}painEP2451) gene; this gene encodes a non-selective cation channel in the TRPA family that is required for the detection of noxious heat and mechanical stimuli. The PBac insertion disrupts the function of the gene, hence, this strain cannot feel noxious stimuli and is not affected by neuropathic pain (Khuong et al., 2019). Thus, their seizure duration should not be affected by the amputation performed to induce chronic neuropathic pain.
It was previously discovered that chronic neuropathic pain results in a reduction of inhibition in the central nervous system (CNS); more specifically, nerve injury brought about via leg amputation causes the death of GABAergic neurons in the ipsilateral and contralateral sections of the ventral nerve cord (VNC) (Khuong et al., 2019). This pain is sensed through Pickpocket-positive (ppk+) sensory neurons and blocking the synaptic output of such neurons prevents GABA loss after amputation (Khuong et al., 2019).
GABA is the main inhibitory neurotransmitter in the CNS that counteracts neuronal excitation to keep network activity balanced in the brain (Allen et al., 2022). It can bind to two receptors that help accomplish this: GABAA and GABAB. GABAA receptors are ionotropic and allow chloride influx in response to ligand binding. Whereas GABAB receptors are metabotropic G-protein coupled receptors; their activity results in increased potassium conductance across neurons, decreased calcium entry, and inhibition of the presynaptic release of other transmitters (Treiman, 2001). It has been documented that a loss or a decreased functionality of GABAergic neurons is seen in epilepsy patients. Neuronal excitation goes unchecked when GABAergic neurotransmission is impaired which can result in seizures. We expected to observe a decrease in the GABAergic neuronal population following the leg amputation (Khuong et al., 2019). We hypothesized that impaired GABAergic neurotransmission would disinhibit neurons, causing network hyper-synchrony and seizures. If there are less inhibitory neurotransmitters, unchecked neuronal signaling will lead to seizures through a similar pathway that increases chronic pain (Khuong et al., 2019). We hypothesized that the leg amputation known to cause neuropathic chronic pain will lead to an increase in seizure duration in seizure prone jus mutants due to a reduction in GABAergic neurons.
Studying the relationship between chronic pain and seizure intensity paves a path for researchers to develop future experiments that can more accurately address the common mechanisms underlying epileptogenesis and neuropathic pain. This research also proposes a mechanism that is involved in pain-induced seizures. This research may lay the groundwork for novel therapeutic strategies, providing treatments to patients suffering from epilepsy or neuropathic pain.
Materials and Methods
Fly Stock
All files in the current study are purchased from Bloomington Drosophila Stock Center (BDSC, Indiana University). After receiving flies from BDSC, they were moved into a new vial containing fresh food and stored at room temperature. The w1118 is the parent fly line for seizure susceptible mutant jus (BDSC# 18817) and painless mutant pain (BDSC# 27895). Flies were maintained using a food mixture consisting of purified water, agar, potassium sodium, calcium chloride, sucrose, dextrose, deactivated yeast, and corn. Methyl benzoate and propanoic acid were added to this mixture to act as an anti-fungal for the flies so that they do not pass down unwanted diseases to their offspring. These were pumped into two to three trays with 100 tubes each and were made every two to three weeks in order to make sure that the flies had fresh food available and are constantly reproducing. The experiments were performed one to two weeks post eclosure on the flies.
Experimental Design
In order to test the hypothesis, we developed and followed an experimental method that allowed us to study the effects on seizures of a leg amputation known to cause chronic pain and GABA loss. This included the utilization of three different D. melanogaster strains: w1118, jus, and pain. About half of the flies of each strain received the experimental treatment (leg amputation), and the rest did not. The control groups included w1118 (n = 30), bang- sensitive jus mutant (n = 30), and painless pain mutant (n= 30) D. melanogaster that were not subjected to the experimental treatment of leg amputation. These groups served as a negative control with the w1118 allowing us to measure the baseline level of seizures, since w1118 is the parental genotype. The mutant jus D. melanogaster allowed us to observe the effects of amputation on specifically seizure prone subjects. These flies best represent those suffering from a convulsive condition due to seizures. The pain mutant that was both amputated and non-amputated served as a negative control. We hypothesized that there would be no significant change in their seizure duration before and after amputation. This mutant was utilized to confirm that the neuropathic pain mechanism was causing seizures through decrease in inhibition caused by the death of GABAergic neurons. The experimental groups included w1118 (n = 19), jus mutant (n = 26), and pain mutant (n = 21) D. melanogaster that were subjected to the leg amputation. The flies separated for amputation underwent a leg-cutting surgery to induce neuropathic chronic pain and were left for a period of 1 week before vortexing. By cutting off one of the back legs, they were subjected to a nerve injury (Khuong et al., 2019). For each vortexing trial, the flies were placed in an empty Petri dish and vortexed for 10 seconds to induce seizures. The process was recorded using an iPhone 13 and the videos were assessed according to the seizure assay to measure the seizure duration in seconds for each fly.
Leg Amputation Assay
The leg amputation assay was adopted from a review of published literature (Khuong et al., 2019) where researchers induced chronic neuropathic pain on D. melanogaster. A modification was made to the published procedure when conducting the leg amputation. Instead of cutting the middle leg, we cut the back leg of the D. melanogaster. The flies had their back leg cut below the femur and above the tibia (Fig. 1). This was accomplished by first moving the flies from their vials to a petri dish. The flies were anesthetized via cold temperature induction as described in the seizure assay. Flies were then placed under a stereomicroscope and micro shears were utilized to amputate their legs. After leg amputation, all flies were returned to their vials and were allowed to rest for one week before being subjected to the vortexing assay. This nerve injury is known to cause chronic pain from the time of amputation until over a week later (Khuong et al., 2019). After one-week, injured flies were placed over a vortexer and the method described in the seizure assay was executed. This was accomplished so we could observe if the seizure duration of these flies was different from flies who were not subjected to the leg amputation.
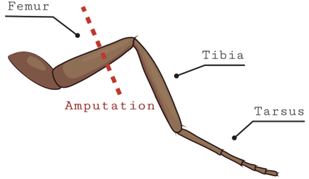
Figure 1. Diagram showing location of the leg amputation in flies. The back leg of the fly (either left or right) was cut between the tibia and femur while they were anesthetized on ice. These flies were then left for a week to rest.
Seizure Assay
We utilized a brief vortex mechanostimulation method to induce seizures. D. melanogaster were moved from their vial of food and placed into a Petri dish in groups of about ten. Flies were moved by placing the vial containing them on an ice pack. The colder temperature anesthetized the flies to make it easier to handle and move them. The vortexing method was accomplished by placing the Petri dish with about ten flies on a vortexer at max speed for ten seconds (Fig. 2). As outlined in previous literature (Parker et al., 2011), D. melanogaster are expected to go through six distinguishable phases of seizure : (1) The introduction of the “bang” (the initial mechanostimulation via vortexing), (2) initial seizure (leg shaking, abdominal muscle contractions, wing flapping, and proboscis extension), (3) initial paralysis stage (immobile and unresponsive to mechanical stimulation), (4) tonicclonic-like activity (stiffening and then twitching/jerking of the muscles), (5) recovery seizure, (6) refractory period (when the organisms cannot have more seizures), (7) recovery period (when flies regain bang-sensitivity).
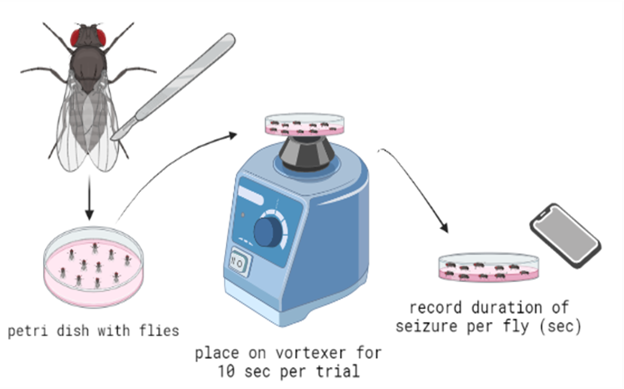
Figure 2. Diagram for the experimental procedure to induce seizures in flies. After the leg amputation, flies were placed into a petri dish, vortexed for 10 seconds per trial, and then removed and placed on a flat surface. These flies were recorded, and the seizure duration of each fly was logged.
Statistical Analysis
We performed a two-way ANOVA with a Tukey post-hoc in R statistical software (v4.1.2; R Core Team 2021) to determine whether there were any significant differences in the seizure duration between the various groups (Table. 1).
Results
To test the hypothesis, we designed an experimental procedure that included the amputation of the back leg of D. melanogaster. The duration of seizure activity was recorded one week after the amputation. Table 1. Two-way ANOVA test results.
df | SS | MS | F- Value |
p – Value | |
Within Group (Strain) | 2 | 76792 | 38396 | 1644.4 | <2e-16 |
Within Group (Amp) | 1 | 4007 | 4007 | 171.6 | <2e-16 |
Between Groups | 2 | 5850 | 2925 | 125.3 | <2e-16 |
Residuals | 150 | 3502 | 23 |
Strain = genotypes of D. melanogaster, amp = amputation, df = degrees of freedom, SS = sum of squares, MS = mean square.
We found that w1118 D. melanogaster with no amputation had a mean (± standard deviation) seizure duration of 5.87 s ± 0.82. A low baseline was expected since the non-amputated w1118 are not already prone to seizures and can be identified as healthy flies. The pain mutant with no amputation had a mean seizure duration of 1.60 s ± 0.77 which was significantly lower than the w1118 baseline (f: p < 0.05, Fig. 3). We hypothesize this is due to its insensitivity to thermal and mechanical stimuli. The jus mutant had a baseline seizure duration of 37.90 s ± 2.20 which was significantly higher than the w1118 flies (e: p < 0.001, Fig. 3) and the pain mutant (c: p < 0.001, Fig. 3). This was expected, as the jus mutant was already prone to seizures before being subjected to the amputation due to its bang-sensitive mutation. Compared to its non-amputated control group, seizure duration of the jus mutant increased to 64.42 s ± 11.32 (a: p < 0.001, Fig. 3) after amputation. However, the w1118 flies and pain mutant did not experience a significant change after the amputation, displaying a seizure duration of 5.05 s ± 2.41 and 3.71 s ± 0.96 respectively.
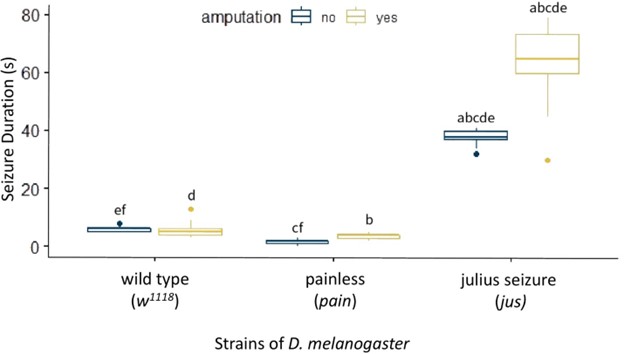
Figure 2. Effect of Amputation on Seizure Duration in D. melanogaster. A box plot measuring seizure duration in seconds on the y-axis and strains of D. melanogaster on the x-axis. These strains are split into two treatments: without amputation (dark blue) and with amputation (yellow). The following groups are formed: w1118 without amputation (n = 30), w1118 with amputation (n = 19), pain mutant without amputation (n = 30), pain mutant with amputation (n = 21), jus mutant without amputation (n = 30), and jus mutant with amputation (n = 26). a, b, c, d, e: p < 0.001, f: p < 0.05. Groups with the same letters are significantly different from each other. The vertical length of the box is the interquartile range, the ends of the whiskers are the maximum and minimum values, the horizontal line inside the box is the median, and the dots are outliers. The baseline Seizure Duration of jus is significantly higher than w1118 and pain, significantly increasing after amputation.
In conclusion, the jus mutant becomes more susceptible to seizures after undergoing a leg amputation, while the w1118 and pain are not affected by it, showing no significant change in their seizure duration before and after amputation. Furthermore, looking specifically at the non-amputated flies, jus mutant is more susceptible to seizures than w1118, while pain mutant is less susceptible than w1118 and jus both.
Discussion
In the current study, we tested a hypothesis that chronic pain may impact the duration of seizure. The data supported the view that a longer seizure duration is associated with a leg amputation that was previously discovered to induce chronic neuropathic pain and loss of GABA. We observed that the jus mutant exhibited a significant increase in seizure duration after being exposed to leg amputation. One possible underlying mechanism as to why this is occurring is due to further loss of GABAergic neurons following the injury.
This specific back leg amputation between the femur and tibia results in the death of GABAergic neurons present in the VNC which leads to over-excitation in the CNS. Leg amputation of D. melanogaster was sufficient to result in lower GABAergic neuronal populations (Khuong et al., 2019). A reduction in GABA producing neurons disturbs the fragile balance of excitation and inhibition in the brain (Allen et al., 2022). This disinhibits neural networks, resulting in hyper- synchronous network activity, which underlies seizures. With a decrease in inhibitory neurons, unchecked excitatory neurotransmitters in the brain are known to cause seizure-like symptoms (Shao et al., 2019). In fact, a reduction of GABAergic functioning is seen in patients with pro-convulsive conditions such as epilepsy (Treiman, 2001). This finding points to the importance of GABAergic neurons in maintaining physiological brain activity. Through our findings, we suggest that a similar mechanism exists behind the effect of neuropathic pain on seizure duration.
Under normal conditions, wild type flies are not prone to seizures. This was supported by the results, which indicated that there was no significant change in seizure duration before and after amputation in w1118 flies. The w1118 flies have a normal level of GABAergic functioning unlike the seizure susceptible jus mutants; hence, we hypothesized that the nerve injury alone should not have a large enough impact on their seizure duration and susceptibility. A possible explanation is that the loss of GABA was insufficient and an already existing impairment in GABA production was required to cause a significant impact on seizure duration. The results supported this hypothesis and allowed us to conclude that healthy subjects are not expected to experience increased seizure activity after an injury.
The use of pain mutant additionally supports the proposed mechanism of GABAergic reduction causing an increase in seizure duration. These flies have an alteration in their DNA sequence by performing a PBac transgenic insertion in the pain gene, disrupting its protein production and preventing the flies from detecting noxious stimuli. We hypothesized that this pain mutant would not be observed to have a greater seizure duration after the amputation. Our reasoning was that since this specific strain cannot detect pain, they should not experience an increase in seizure duration after the nerve injury. Once again, our hypothesis was supported through our observations since the pain mutant did not have an increase in seizure duration after the amputation. A significantly decreased seizure duration was seen in pain non-amputated mutants compared to w1118 non- amputated flies. A possible explanation for this could be that since these flies are insensitive to heat and mechanical stimuli, they were not affected by the seizure and leg-cutting assay on the same level as the w1118 flies. As the differences in seizure duration of amputated w1118 and pain flies were not significant, comparisons between the two groups do not prove fruitful.
As for the jus mutant, leg amputation had a significant effect on increasing seizure susceptibility (Fig. 3). The jus mutant was chosen to model epilepsy since the GABAergic functioning of the jus mutant was already impaired; this is why seizures were observed even before the induction of the treatment method. We hypothesized that nerve injury would cause further loss of GABAergic functioning; this hypothesis was supported by the results since longer seizure durations were observed after the leg cutting assay was used on the bang-sensitive mutant.
There’s a possibility that the results of these trials may have alternate interpretations as well. The leg cutting assay may have had unexpected effects on fly behavior. For example, if chronic neuropathic pain led to a heightened stress response in the D. melanogaster, there is a chance that stress influenced the observed results as preclinical research indicates that stress may lead to heightened seizure responses (Espinosa-Garcia et al., 2021). Additionally, D. melanogaster utilized in this study have unique diurnal activity levels that should be noted. It has been observed that D. melanogaster have lower locomotive activity levels during the midday hours. (Cao et al., 2017). This leads to flies being less responsive when stressed during these hours, which is when we performed the experiments.
Other possible sources of error leading to the conclusion would be that the video analysis of flies when they were experiencing seizures may have been incorrectly interpreted due to human error and lack of advanced technology, leading to inaccurate seizure duration data. During transfer of videos to other devices for analysis, a decrease in resolution occurred as well, causing further difficulty with analyzing the seizure duration. Another source of error is that the D. melanogaster were not the same age and were born at different times; this may have an effect on pain levels experienced. It would also be important in replicating results to pay attention to pregnancy and leave any pregnant flies out of the testing groups in order to ensure an equal brain chemistry throughout all the flies being tested. Furthermore, the time of day when we tested these flies could affect our results. D. melanogaster tend to sleep during the middle of the day (afternoon), which is the time that we were running these experiments. This could affect their locomotive behavior and result in observations that could change depending on the time of day. Lastly, amputating the middle leg instead of the back leg is ideal when attempting to maximize neuropathic pain (Khuong et al., 2019). Although the leg cutting assay was designed for the amputation of the middle leg, the back legs were cut instead.
For future continuations of this study, a possible experimental design would be to breed together the seizure prone jus and pain mutants to produce offspring that do not sense pain but are also prone to seizures. Performing the leg amputation would introduce them to neuropathic pain, but they should not be able to sense pain. After performing the seizure (vortex) assay on these injured flies, we will be able to observe their seizure duration and determine if there is a significant change before and after leg amputation. The results of this experiment will allow us to further improve the knowledge on how chronic neuropathic pain affects seizure duration in seizure prone subjects. This proposed experiment would help prove that an increase in seizure duration is due to the loss of GABAergic neurons, and not from neuronal pain signaling.
Millions of people around the world suffer from chronic pain and pro-convulsive conditions. Many of these diseases, like epilepsy, can be resistant to drugs or therapies that attempt to treat symptoms such as seizures. While there is much clinical evidence and research surrounding the treatment of epilepsy and chronic pain conditions, this is one of the first studies that attempts to draw a link between the two. We observed that chronic neuropathic pain produced greater seizure intensities and durations in D. melanogaster. This observation led us to propose that a reduction in GABAergic functioning is the underlying mechanism that is causing this difference. There is hope that new medication and treatments can be developed that target this mechanism, while future research attempts to study this phenomenon in detail. Advancing knowledge on the connection between chronic neuropathic pain and seizures could provide relief for millions around the world.
Acknowledgments
We would like to thank Dr. Kwangwon Lee and Dr. Nathan T. Fried for their continued support and constant mentorship, as well as Christina Souto for her preliminary work on adopting the seizure assay to this experiment. We would also like to thank Rutgers Camden Biology Department for their facilities.
Refrences
Arout, C.A., Sofuoglu, M., Bastian, L.A., and Rosenheck, R.A. (2018). Gender Differences in the Prevalence of Fibromyalgia and in Concomitant Medical and Psychiatric Disorders: A National Veterans Health Administration Study. Journal of Women’s Health 27, 1035–1044. 10.1089/jwh.2017.6622.
Allen, M.J., Sabir, S., Sharma, S. (2022) GABA Receptor. In: StatPearls [Internet]. Treasure Island (FL):
StatPearls Publishing https://www.ncbi.nlm.nih.gov/books/NBK526124/
Cao, W., and Edery, I. (2017). Mid-day siesta in natural populations of D. melanogaster from Africa exhibits an altitudinal cline and is regulated by splicing of a thermosensitive intron in the period clock gene. BMC Evolutionary Biology 17, 32. 10.1186/s12862-017-0880-8.
https://bmcecolevol.biomedcentral.com/articles/10.1186/s12862-017-0880-8
Curing the Epilepsies: The Promise of Research | National Institute of Neurological Disorders and Stroke https://www.ninds.nih.gov/current-research/focus-disorders/focus-epilepsy-research/curing-epilepsiespromise-research.
Does an exploding brain network cause chronic pain? — ScienceDaily https://www.sciencedaily.com/releases/2018/01/180112132916.htm.
Espinosa-Garcia C., Zeleke H., Rojas A. (2021). Impact of Stress on Epilepsy: Focus on
Neuroinflammation-A Mini Review. Int J Mol Sci. 22(8):4061. 10.3390/ijms22084061
Fibromyalgia – Symptoms and causes Mayo Clinic. https://www.mayoclinic.org/diseasesconditions/fibromyalgia/symptoms-causes/syc-20354780.
Fly Base Gene Report: Dmel\jus FBgn0039647. (2023). Fly Base FB2023_01. https://flybase.org/reports/FBgn0039647.html
Goleva, S.B., Lake, A.M., Torstenson, E.S., Haas, K.F., and Davis, L.K. (2020). Epidemiology of Functional Seizures Among Adults Treated at a University Hospital. JAMA Netw Open 3, e2027920.
10.1001/jamanetworkopen.2020.27920.
He, J., Li, B., Han, S., Zhang, Y., Liu, K., Yi, S., Liu, Y., and Xiu, M. (2022). Drosophila as a Model to Study the Mechanism of Nociception. Frontiers in Physiology 13.
Horne, M., Krebushevski, K., Wells, A., Tunio, N., Jarvis, C., Francisco, G., Geiss, J., Recknagel, A., and Deitcher, D.L. (2017). julius seizure, a Drosophila Mutant, Defines a Neuronal Population Underlying Epileptogenesis. Genetics 205, 1261–1269. 10.1534/genetics.116.199083.
Karpova, E.K., Eremina, M.A., Pirozhkova, D.S., and Gruntenko, N.E. (2019). Stress-related hormones affect carbohydrate metabolism in Drosophila females. Arch Insect Biochem Physiol 101, e21540. https://doi.org/10.1002/arch.21540.
Keogh, E., McCracken, L.M., and Eccleston, C. (2005). Do men and women differ in their response to interdisciplinary chronic pain management? Pain 114, 37–46. https://doi.org/10.1016/j.pain.2004.12.009.
Khuong, T.M., Wang, Q.-P., Manion, J., Oyston, L.J., Lau, M.-T., Towler, H., Lin, Y.Q., and Neely, G.G. (2019). Nerve injury drives a heightened state of vigilance and neuropathic sensitization in Drosophila. Sci Adv 5, eaaw4099. 10.1126/sciadv.aaw4099.
Lee, J., and Wu, C.-F. (2002). Electroconvulsive Seizure Behavior in Drosophila: Analysis of the Physiological Repertoire Underlying a Stereotyped Action Pattern in Bang-Sensitive Mutants. J. Neurosci. 22, 11065–11079. 10.1523/JNEUROSCI.22-24-11065.2002.
Lee, S. (2013). Pharmacological Inhibition of Voltage-gated Ca2+ Channels for Chronic Pain Relief. Current Neuropharmacology 11, 606–620.
Liu, L.-Y., Zhang, R.-L., Chen, L., Zhao, H.-Y., Cai, J., Wang, J.-K., Guo, D.-Q., Cui, Y.-J., and Xing, G.-G. (2019). Chronic stress increases pain sensitivity via activation of the rACC-BLA pathway in rats. Exp Neurol 313, 109–123. 10.1016/j.expneurol.2018.12.009.
Ly, C.V., Yao, C.-K., Verstreken, P., Ohyama, T., and Bellen, H.J. (2008). straightjacket is required for the synaptic stabilization of cacophony, a voltage-gated calcium channel α1 subunit. J Cell Biol 181, 157–170. 10.1083/jcb.200712152.
Munce, S.E.P., and Stewart, D.E. (2007). Gender differences in depression and chronic pain conditions in a national epidemiologic survey. Psychosomatics 48, 394–399. 10.1176/appi.psy.48.5.394.
Parker, L., Howlett, I.C., Rusan, Z.M., and Tanouye, M.A. (2011). SEIZURE AND EPILEPSY: STUDIES OF SEIZURE DISORDERS IN DROSOPHILA. Int Rev Neurobiol 99, 1–21. 10.1016/B9780-12-387003-2.00001-X.
Pulver, S.R., and Berni, J. (2012). The Fundamentals of Flying: Simple and Inexpensive Strategies for Employing Drosophila Genetics in Neuroscience Teaching Laboratories. J Undergrad Neurosci Educ 11, A139–A148.
R Core Team (2021). R: A language and environment for statistical computing. R Foundation for Statistical Computing, Vienna, Austria. URL https://www.R-project.org/.
Rasker, J.J., Wolfe, F., Klaver-Krol, E.G., Zwarts, M.J., and ten Klooster, P.M. (2021). The relation of fibromyalgia and fibromyalgia symptoms to self-reported seizures. PLoS One 16, e0246051.
10.1371/journal.pone.0246051.
Shao, L.R., Habela, C.W., Stafstrom, C.E. (2019) Pediatric Epilepsy Mechanisms: Expanding the Paradigm of Excitation/Inhibition Imbalance. Children (Basel). 6(2):23. doi: 10.3390/children6020023. PMID: 30764523; PMCID: PMC6406372.
Sokabe, T., Tsujiuchi, S., Kadowaki, T., and Tominaga, M. (2008). Drosophila Painless Is a Ca2+Requiring Channel Activated by Noxious Heat. J. Neurosci. 28, 9929–9938. 10.1523/JNEUROSCI.2757-
08.2008.
Tatum, W.O., Langston, M.E., and Acton, E.K. (2016). Fibromyalgia and seizures. Epileptic Disord 18, 148–154. 10.1684/epd.2016.0823.
Treiman, D.M. (2001). GABAergic mechanisms in epilepsy. Epilepsia 42 Suppl 3, 8–12. 10.1046/j.15281157.2001.042suppl.3008.x.
Zdraveska, N., and Kostovski, A. (2010). Epilepsy presenting only with severe abdominal pain. J Pediatr Neurosci 5, 169–170. 10.4103/1817-1745.76123.
Journal of Biological Sciences at Rutgers Camden (JBS) is licensed under a Creative Commons Attribution-NonCommercial-ShareAlike 4.0 International License