Blessing Awogbamila, Guddi Brahmbhatt, Jenna Brodnyan, Paula Khoiniha, Rosemarie Arroyo-Martinez
Department of Biology, Rutgers University, Camden NJ 08102
Abstract
Globally, drought is a major agricultural and economic problem, specifically due to its effects on crop yield. Effective and economical strategies to mitigate drought stress in agricultural plants must be developed as drought continues to escalate in both frequency and intensity. Substances known as biostimulants have been studied for their agricultural outputs, as they are capable of increasing nutrient uptake in plants, enhancing crop quality traits, and increasing tolerance of abiotic stress, such as drought. Currently, commercially produced biostimulants are expensive. A cost-effective alternative to commercial biostimulants is honey, which is considered a natural biostimulant by-product of sustainable agriculture. Honey has been shown to be beneficial when sprayed directly onto the leaves (i.e., the foliar method) of crops that are experiencing abiotic stress, such as drought. However, the precise study of drought mitigation using diluted honey has not been studied using the dicot model plant Arabidopsis thaliana or by using the subirrigation method. In this study, we hypothesized that exogenous diluted honey reduces the effects of drought stress on A. thaliana, and its beneficial effects are due to the biostimulants in honey. To test this hypothesis, plants exposed to drought and normal conditions were administered various concentrations of honey, phosphate solution, and water. Seed volume and biomass, which are measures of plant yield, were evaluated and recorded. We found that diluted honey reduced crop lifespan while maintaining or increasing crop yield when applied by the subirrigation method to plants under both normal and drought conditions. Additionally, diluted honey increased seed size in plants subjected to drought stress, but it does not affect the seed volume of plants under normal and drought conditions. Given that the diluted honey treatments produced the same biomass in less time than the control groups under drought stress, our results supported the view that application of diluted honey by subirrigation may prove to be a sustainable, naturally available, and economically advantageous agricultural practice to overcome drought stress.
Introduction
Drought has long been a major concern when it comes to crop yield. A prolonged dry period, known as a drought, develops slowly and is characterized by a lack of precipitation that causes water scarcity due to a lack of rainfall. Higher temperatures, a result of global warming, have resulted in increases in soil evaporation. Consequently, single and multi-year droughts have been increasing in frequency across the United States of America (US). Therefore, in order to prevent severe drought events, more feasible, economical, and efficient alternatives must be devised. One viable solution to the impact of drought on agriculture is the use of biostimulants.
When a community is affected by drought, the effects can be disastrous. Decreased food production and decreased water supply and quality are just a few of these effects that can cause an increase in health risks to people. These effects can be extensive, intricate, and expensive. An estimated 55 million people globally are affected by droughts every year (Stanke et al. 2013). Droughts are among the most costly natural catastrophes. Over 41 years (1980–2021), there were 29 drought events recorded in the US that caused severe economic impact, totaling $285.4 billion USD and loss of life, resulting in 4139 deaths (Jalalzadeh Fard et al. 2022). Based on the Palmer Drought Index, severe to extreme drought affected approximately 27% of the contiguous US as of the end of October 2022 (NCEI 2022). In the state of California alone, the current western drought has cost over $4.9 billion thus far and has contributed to the loss of more than 21,000 jobs.
Biostimulants are informally defined as any microorganism or substance applied to plants that increase the efficacy of nutrient uptake, enhance tolerance against abiotic stresses, and/or enhance crop quality traits (du Jardin 2015). Emphasis is placed on “informally defined” because specific framework regarding such substances is lacking in the US and, consequently, there is little regulation. Biostimulants are widely defined by having agricultural outputs, such as effects on drought or salinity tolerance. Those containing phytohormones have been noted to play a key role in regulating plant physiological behaviors, such as seed germination or protecting plants from abiotic cues (Fahad et al. 2015). The challenge is that many commercially produced biostimulants, such as antioxidants or phytohormones, are expensive. In addition to a formal definition, proper regulation and obtaining biostimulants through more economical sources is critical. Some naturally occurring substances, such as plant extracts and bee honey, contain essential components – particularly phytohormones and osmoprotectants – that shield plants from abiotic stress. The effects of exogenous diluted bee honey on model species A. thaliana after a period of drought stress will be studied in this experiment in order to increase our knowledge of honey’s capabilities as a biostimulant.
Bee honey in particular contains osmoprotectants, mineral nutrients, and vitamins that take on the role of antioxidants, all of which also contribute to promote plant growth; it has proteins, enzymes, and amino acids as well as fatty acids that are beneficial to plants (Semida et al. 2019). Honey also contains a variety of phytohormones, a group of organic substances that occur naturally, which help in regulating plant growth in low concentrations (Wang et al. 2016). These phytohormones in honey, such as abscisic acid and salicylic acid, have also been shown to promote resistance to drought stress in plants (Iqbal et al. 2022). Abscisic acid has been found to contribute in regulating stomatal closure, keeping up osmolyte synthesis, and helping with gene upregulation (Iqbal et al. 2022). Salicylic acid will start being produced in response to drought stress and will accumulate in order to benefit the plant. It responds to drought stress with its antioxidant activity and protects photosynthetic machinery, leading to protection against electron leakage (Iqbal et al. 2022). Diluted concentrations of bee honey have been used before to study its effects on plants that have been subjected to stress. It has mitigated the effects of drought stress on cell membrane stability as well as ion-leakage, helping with water content in plants that were placed under drought stress conditions. It had positive effects on crops such as onions (Semida et al. 2019), monocotyledons, and chili peppers (Semida et al. 2019), dicotyledons. This is especially relevant since the model of interest in this project is A. thaliana, a dicot. Quantifying the effects of raw bee honey on A. thaliana’s tolerance to drought stress is necessary as the effects of honey as a biostimulant on the model plant still needs to be addressed in the scientific literature.
A. thaliana is a model plant that can grow under simple laboratory conditions. It became an essential model organism because of the availability of various information and tools, such as whole genome sequence, molecular genetic markers and large collections of sequence-indexed DNA-insertion mutants, in addition to the ease of generating transgenic plants (Hayashi and Nishimura 2006). It offers a window into the molecular, cellular, and developmental mechanisms underlying life as a multicellular photoautotroph (Woodward and Bartel 2018). Also, its small size and simple growth requirements make it easy to grow in laboratory conditions. It has a short life cycle of ~8 weeks and is a self-fertilizing diploid that produces thousands of seeds from one individual (Somerville and Koornneef 2002).
In this study, seed count and biomass were measured to assess drought tolerance. The primary determinant of the economic feasibility of grain agricultural crops is seed yield, hence the main method for estimating seed yield is by assessing the biomass and seed count parameters. Additionally, detailed phenotypic information for numerous mutants affecting seed parameters and biomass is available, making seed count and biomass good parameters to include for future studies (Van Daele et al. 2012). Wheat establishment is known to require biomass during the early vegetative stage, particularly in regions with insufficient water availability. Good wheat establishment requires shoots to emerge from the soil and to develop leaf area as quickly as possible, which would allow the plants to increase their competitiveness and reduce loss of water and nutrients (Kaya 2016). A significant benefit in agricultural applications would be the ability to maintain a typical biomass (i.e., plant biomass under normal conditions) while under drought conditions. As a conclusion, in this experiment, biomass is measured for each plant that is exposed to both normal and drought conditions.
Due to an abundance of phytohormones, antioxidants, and antibacterial characteristics in honey, it is anticipated that honey application will enhance the resistance to drought stress. Our data supports the hypothesis that diluted raw clover honey would act as a biostimulant for A. thaliana under drought conditions. Given this data, honey could be a good option for an inexpensive and more widely available alternative to synthetically produced biostimulants.
Materials & Methods
A. thaliana Culture
Seeds were sown in Murashige and Skoog (MS) media, which was made with 7.5 g Bacto Agar and 1 L MS solution. This was autoclaved on the liquid cycle for 25 minutes and cooled to 60 °C. In the sterile hood, 1 mL Gamborg’s vitamins were added. After the seeds were sown in the media, they were placed in a 4 °C fridge for 48 hours. After this, the media containing the seeds were placed in the room with the below indicated conditions for about 12 days to germinate, then they were transferred to the below described pots.
For initial growth and development of A. thaliana prior to inducing drought conditions, plants were grown under 5000 Lux light intensity in a 16 hour light/8 hour dark cycle at a constant room temperature of 22 °C. The plants were grown in a mixture of two parts soil, one part perlite, and one part vermiculite. Plants were sub-irrigated (1 cm of water from bottom of tray) every three days for the first two weeks. By the end of the two weeks, most of the plants began to sprout, which was earlier than expected. Due to this, drought conditions were induced on three (3) pots of each experimental group after the end of the two weeks.
Experimental Design
Four different groups of plants were assessed in this experiment. The first and second groups were designated as the plants that were administered 10 g/L and 20 g/L honey, respectively, after experiencing drought conditions. The third group was designated as the plants that were administered 0.75 g/L phosphate solution (e.g., Miracle Gro) after drought conditions, which would serve as the positive control. Phosphate fertilizer has been studied in the past for its positive effect on drought tolerance of plants. The fourth group included the plants that were given water after experiencing drought conditions, which would serve as the negative control. Each group included three pots that underwent drought stress and three pots that experienced normal conditions. Each pot held four plants. Normal conditions involved regular watering, whereas drought conditions involved a lack of watering. The seed count, biomass, lifespan, and seed size would be evaluated after treatment was completed.
Drought Assay
Drought conditions were induced by not watering the plants until the effects of drought began to present themselves phenotypically, namely by loss of cell turgor (i.e., wilting), rate of cell senescence and drooping, leaf rolling and brittleness, and leaf yellowing. It took nine (9) days for these phenotypic signs to occur. After experiencing drought stress, plants were selected and grouped according to their similarities in primary shoot height before inducing experimental conditions.
Both the honey and Miracle Gro were diluted with water and plants were watered with the experimental treatments by the sub-irrigation method as needed (i.e., when the solutions were completely uptaken by the plants in each tray) for one week. This ended up being about 500 mL of treatment every other day. This method was employed to keep a consistent watering technique among the treatment groups.
Seeds were collected using the “hand threshing method” (Rivero et al. 2014). A few white papers were placed on the bench for collection of the threshed seeds. Plants were cut from right above the soil, then dry plants were crushed, one by one, using hands to remove all the seeds from siliques over a clean sieve placed on top of the white paper. The seeds were sifted through the mesh until they were free of chaff. Each pot contained four plants, and each pot is considered one experimental unit. The seeds of all the plants in one pot were combined into one graduated Eppendorf tube. After collecting the seeds, the remaining plant material consisting of the shoot system was placed in a sealed plastic bag until they were dry. Plant material of the same pots (1 pot = 4 plants) were put into the same bags and seeds of the same pots were placed into the same Eppendorf tubes.
Seed size was measured by placing approximately 10 microliters of seeds from each treatment group under drought conditions into a Petri dish and taking images with a microscope. After the images were taken, they were evaluated using ImageJ (National Institutes of Health). Due to there being a large difference in seed count for each 10 microliter sample, a random sample of 200 seed measurements were taken from each treatment group and the average was found. The statistical test that was performed is the two-way ANOVA using GraphPad Prism (Dotmatics). Each experimental group was compared to the negative control experiencing normal and drought conditions.
Statistical Analyses
The statistical test that was performed is the two-way ANOVA using GraphPad Prism (Dotmatics). Each experimental group was compared to the negative control experiencing normal and drought conditions.
Results
To test our hypothesis, we grew A. thaliana under normal conditions (regular watering using the subirrigation method). Once the plants began to sprout, we put half of them under drought conditions (no watering) and kept half under normal conditions. Once phenotypic signs of drought presented themselves, we placed three (3) pots that had been under normal conditions and three (3) pots that had been under drought conditions into one tray. There were four (4) trays in total – two (2) experimental groups and two (2) control groups. The trays were then given their respective treatments (20 g/L honey, 10 g/L honey, phosphate solution, water) for one week using the subirrigtion method. Biomass, seed volume, seed size, and lifespan were the parameters used to determine the viability of bee honey as a biostimulant.
The biomass was found by weighing the shoot system of the plants after removing the siliques for seed collection and allowing the plant material to dry. To determine if there was a significant difference in biomass, the average weight of each pot from each of the four experimental groups: Honey 10 g/L, Honey 20 g/L, Phosphate, and Water, were compared to each other under both normal and drought conditions (Fig. 1A). No significant difference was found when comparing Honey 10 g/L (0.193 +/- 0.040) to Phosphate (0.153 +/- 0.055) or Honey 10 g/L to Water (0.173 +/- 0.084) under drought conditions (2- way ANOVA, p > 0.05) (Fig. 1A). A significant difference was also not found between Honey 20 g/L (0.267 +/- 0.071) and Phosphate or Honey 20 g/L and Water under drought conditions (2-way ANOVA, p > 0.05) (Fig. 1A). the experimental and control groups under drought conditions. A comparison of Honey 10 g/L (0.383 +/- 0.057) and Water (0.220 +/- 0.053) under normal conditions was found to be statistically significant, indicating that the addition of diluted bee honey contributes to a larger biomass (2-way ANOVA, p < 0.05) (Fig. 1A). A comparison between Honey 20 g/L (0.363 +/- 0.072) and Water under normal conditions was also found to be statistically significant (2-way ANOVA, p < 0.01) (Fig. 1A).

Figure 1: (A) This represents the average dried biomass of A. thaliana across normal and drought conditions with applied treatments. Honey 20 g/L compared to Water experiencing normal conditions was statistically significant (2-way ANOVA, p < 0.01) as well as Honey 10 g/L compared to Water experiencing normal conditions (2-way ANOVA, p < 0.05). (B) Represents the seed size of A. thaliana in drought conditions upon treatment. All groups were statistically significant (One-way ANOVA, p < 0.05). (C) This data was derived from measuring the volume of the seeds (0.1 mL = 625 seeds) from plants experiencing drought stress. All groups were insignificant. (D) Represents the lifespan of A. thaliana experiencing treatments. Honey treatment groups had a significantly shorter lifespan than both control groups (One-way ANOVA, p < 0.0001). Note: (****, p < 0.0001), (***, p < 0.001), (**, p < 0.01), and (*, p < 0.05).
The seed size was found by evaluating the seeds using microscopic photos on ImageJ (Fig. 2). To determine if there was a significant difference in seed size, the average measurement of a 200-unit randomized sample from each of the four experimental groups: Honey 10 g/L, Honey 20 g/L, Phosphate, and Water, were compared to each other under drought conditions (Fig. 1B). A statistical significance was determined when comparing Honey 10 g/L (0.288 +/- 0.085) to Phosphate (0.267 +/- 0.079) and Honey 10 g/L to Water (0.236 +/- 0.078) under drought conditions, indicating that the application of diluted bee honey increases seed size (One-way ANOVA, p < 0.05) (Fig. 1B). A significant difference was also found when comparing Honey 20 g/L (0.302 +/- 0.059) to Phosphate and 20 g/L Honey to Water under drought conditions (One-way ANOVA, p < 0.05) (Fig. 1B).
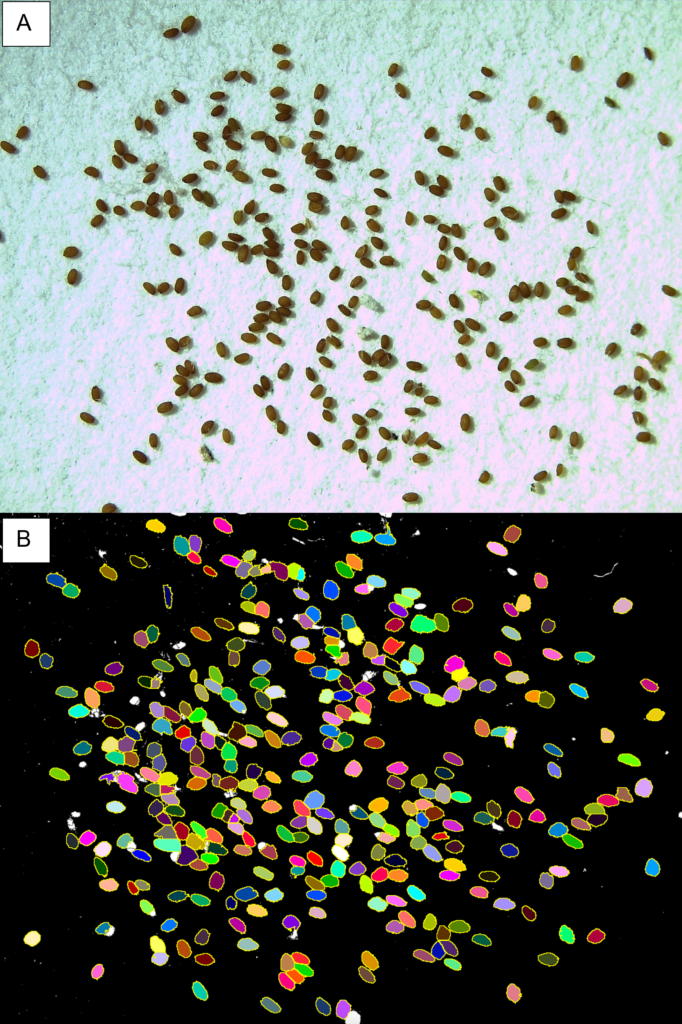
Figure 2: ImageJ was used to quantify the average size of seeds in a 10 microliter sample. (A) An image taken of seeds from the Honey 10 g/L group that had experienced drought conditions. (B) Same image after being processed in ImageJ to assess average seed size.
The seed volume was found by removing seeds from the siliques using the “hand threshing method”, and placing the seeds into a graduated Eppendorf tube. To determine if there was a significant difference in seed volume, the total volume of seeds from each of the four experimental groups: Honey 10 g/L, Honey 20 g/L, Phosphate, and Water, were compared to one other under both normal and drought conditions (Fig. 1C). There was no significance between the four groups when evaluating seed volume under both normal and drought conditions (2-way ANOVA, p > 0.05).
The lifespan was found by recording the time between life and death of the plants. All of the plants’ lives began at the same time. The Honey 20 g/L and Honey 10 g/L groups died within 1-2 days of one another. The Phosphate and Water groups died within 1-2 days of one another, but they both outlasted the honey groups by approximately 20 days. (Fig. 1D). Honey 10 (g/L) (40.3 +/-1.2) and Honey 20 (g/L) (40.5 +/-1.049) had significantly shorter lifespans than the control groups of Water (60 +/- 1.265) and Phosphate (60.5 +/- 1.265) (One-way ANOVA, p < 0.05). This indicates that diluted bee honey decreases the lifespan of plants.
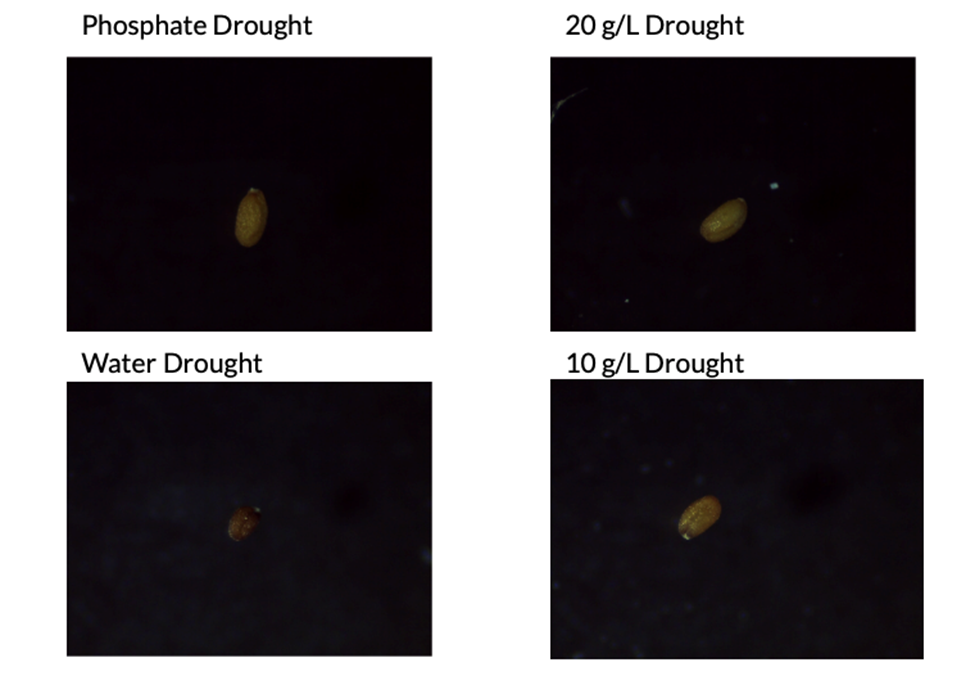
Figure 3: Microscopic images of individual seeds. Fungal contamination was originally a concern, but is not seen here due to lack of white substrate.
Discussion
Previous studies concentrated on the effects of drought on people globally, as well as the mitigation of damage done to crops through the use of exogenously applied substances known as biostimulants. This experiment uses two different diluted concentrations of bee honey as a biostimulant in order to mitigate the effects of drought stress on the dicot model plant A. thaliana. It was discovered that diluted bee honey, when applied to plants via subirrigation under both normal and drought conditions, decreased crop lifespan while preserving or increasing crop yield (i.e., biomass). In addition, diluted bee honey increased the seed size of plants that had experienced drought stress, but it had no effect on the volume of seeds produced by plants in both normal and drought conditions. While the diluted bee honey treatment groups and control groups generated similar values of biomass under drought conditions, treatment groups generated this amount of biomass in a shorter amount of time. Under normal conditions, honey statistically increased biomass, but not under drought conditions, indicating that it appears to enhance plant growth in that area under normal conditions, but not under abiotic stress. However, under both drought and normal conditions, there were statistically significant differences in seed size and lifespan, demonstrating that honey does affect the performance of the plant in various areas.
Under normal conditions, biomass was statistically significant when compared between the experimental (Honey 20 g/L, Honey 10 g/L) and control groups (Phosphate, Water). However, biomass showed statistical insignificance when compared between the experimental and control groups under drought conditions. This indicates that the application of diluted bee honey increases crop yield under normal conditions. However, diluted bee honey may not enhance drought tolerance in the form of increasing crop yield when applied to plants under abiotic stress. It should be noted that while the diluted bee honey treatment groups and control groups generated similar values of biomass while experiencing drought conditions, treatment groups generated this amount of biomass in a shorter amount of time, as evidenced by the treatment groups’ shorter lifespan (Fig. 1D).
ImageJ was utilized to quantify seed sizes. It was found that there were statistically significant differences between the honey treatment groups and both the negative control (Water) and positive control (Phosphate) groups. The only instance in which there was no statistically significant difference was between the honey treatment groups that received 20 g/L and 10 g/L of diluted honey. The sample size varied between the treatment groups due to the larger seed sizes. Random sampling was used to streamline seed size data while avoiding any biases. The size of the seed can be a fitness indication. Larger seeds have historically been reported to be associated with higher levels of fitness (Giles 1990). This occurs because larger seeds typically have higher nutrient contents, which provide them more consistent vigor in the early stages of their life cycle (Giles 1990). The honey treatment groups may have higher fitness, as evidenced by the larger seeds they produced as a result, but this is slightly outside the purview of this study.
The seed volume could be explained by considering the seed sizes. The control groups may have produced more seeds of smaller size, while the treatment groups produced less seeds of larger size. This is supported when contrasting the size of each group’s seeds during periods of drought. In comparison to the control groups, the diluted bee honey treatment groups produced statistically significantly larger seed sizes. This could mean that diluted bee honey vitalizes seed health, thereby causing A. thaliana to produce a smaller amount of larger seeds. In order to experimentally quantify their fitness and correctly compare them with both of the control groups, another experiment in which the seeds that were developed in this experiment are tested for their germination will need to be conducted. Despite the fact that our findings indicated that diluted bee honey shortened crop lifespan while maintaining or even boosting crop yield, such as biomass, when applied to plants using the subirrigation method in both normal and drought conditions. Previous research has demonstrated the benefits of applying diluted bee honey via foliar application to Allium cepa L. (Semida et al. 2019) every 30 days and to Capsicum frutescens L. (Abou-Sreea et al. 2021). Bee honey applied by foliar method to plants may have accelerated nutrient absorption and enabled for prompt nutrient deficits in plants to be remedied (McCall 1980). The drought resistance of A. thaliana was not considerably affected by the sub-irrigation strategy, maybe because it did not allow the nutrients to be absorbed as well. It is necessary to conduct additional experiments using the foliar spray administration approach in order to determine if these are the likely causes of the outcomes of our experiment.
References
Abou-Sreea A. I. B., Azzam C. R., Al-Taweel S. K., Abdel-Aziz R. M., Belal H. E. E., Rady M. M., Abdel-Kader A. A. S., Majrashi A., and Khaled K. A. M. (2021). Natural Biostimulant Attenuates Salinity Stress Effects in Chili Pepper by Remodeling Antioxidant, Ion, and Phytohormone Balances, and Augments Gene Expression. Plants (Basel, Switzerland) 10,. doi:10.3390/plants10112316
Fahad S., Hussain S., Bano A., Saud S., Hassan S., Shan D., Khan F. A., Khan F., Chen Y., Wu C., Tabassum M. A., Chun M. X., Afzal M., Jan A., Jan M. T., and Huang J. (2015). Potential role of phytohormones and plant growth-promoting rhizobacteria in abiotic stresses: consequences for changing environment. Environ Sci Pollut Res 22, 4907–4921. doi:10.1007/s11356-014-3754-2
Giles B. E. (1990). The effects of variation in seed size on growth and reproduction in the wild barley Hordeum vulgare ssp. spontaneum. Heredity 64, 239–250. doi:10.1038/hdy.1990.29
Hayashi M., and Nishimura M. (2006). Arabidopsis thaliana—A model organism to study plant peroxisomes. Biochimica et Biophysica Acta (BBA) – Molecular Cell Research 1763, 1382–1391. doi:10.1016/j.bbamcr.2006.08.014
Iqbal S., Wang X., Mubeen I., Kamran M., Kanwal I., Diaz G., Abbas A., Parveen A., Atiq M., Alshaya H., Zin El-Abedin T., and Fahad S. (2022). Frontiers | Phytohormones Trigger Drought Tolerance in Crop Plants: Outlook and Future Perspectives. Frontiers. https://www.frontiersin.org/articles/10.3389/fpls.2021.799318/full
Jalalzadeh Fard B., Puvvula J., and Bell J. E. (2022). Evaluating Changes in Health Risk from Drought over the Contiguous United States. Int J Environ Res Public Health 19, 4628. doi:10.3390/ijerph19084628
du Jardin P. (2015). Plant biostimulants: Definition, concept, main categories and regulation – ScienceDirect. ScienceDirect. https://www.sciencedirect.com/science/article/pii/S0304423815301850
Kaya Y. (2016). Biomass as a Selection Criterion for Drought Tolerance in Wheat. ResearchGate. https://www.researchgate.net/publication/303494956_Biomass_as_a_Selection_Criterion_for_Drought_Tolerance_in_Wheat
McCall W. W. (1980). Foliar Application of Fertilizers. http://hdl.handle.net/10125/7801
NCEI (2022). October 2022 Drought Report. https://www.ncdc.noaa.gov/sotc/drought/202210
Rivero L., Scholl R., Holomuzki N., Crist D., Grotewold E., and Brkljacic J. (2014). Handling Arabidopsis Plants: Growth, Preservation of Seeds, Transformation, and Genetic Crosses. ‘Arabidopsis Protocols’. (Eds JJ Sanchez-Serrano and J Salinas) Methods in Molecular Biology. pp. 3–25. (Humana Press: Totowa, NJ) doi:10.1007/978-1-62703-580-4_1
Semida W. M., Abd El-Mageed T. A., Hemida K., and Rady M. M. (2019). Natural bee-honey based biostimulants confer salt tolerance in onion via modulation of the antioxidant defence system. The Journal of Horticultural Science and Biotechnology 94, 632–642. doi:10.1080/14620316.2019.1592711
Somerville C., and Koornneef M. (2002). A fortunate choice: the history of Arabidopsis as a model plant. Nat Rev Genet 3, 883–889. doi:10.1038/nrg927
Stanke C., Kerac M., Prudhomme C., Medlock J., and Murray V. (2013). Health Effects of Drought: a Systematic Review of the Evidence. PLoS Curr 5, ecurrents.dis.7a2cee9e980f91ad7697b570bcc4b004. doi:10.1371/currents.dis.7a2cee9e980f91ad7697b570bcc4b004
Van Daele I., Gonzalez N., Vercauteren I., de Smet L., Inzé D., Roldán-Ruiz I., and Vuylsteke M. (2012). A comparative study of seed yield parameters in Arabidopsis thaliana mutants and transgenics. Plant Biotechnology Journal 10, 488–500. doi:10.1111/j.1467-7652.2012.00687.x
Wang Q., Cai W.-J., Yu L., Ding J., and Feng Y.-Q. (2016). Comprehensive Profiling of Phytohormones in Honey by Sequential Liquid–Liquid Extraction Coupled with Liquid Chromatography–Mass Spectrometry | Journal of Agricultural and Food Chemistry. ACS Publications. https://pubs.acs.org/doi/full/10.1021/acs.jafc.6b04234
Woodward A. W., and Bartel B. (2018). Biology in Bloom: A Primer on the Arabidopsis thaliana Model System. Genetics 208, 1337–1349. doi:10.1534/genetics.118.300755
Journal of Biological Sciences at Rutgers Camden (JBS) is licensed under a Creative Commons Attribution-NonCommercial-ShareAlike 4.0 International License