Abstract
The effects greenhouse gases have on the earth, fluctuating temperatures and ocean salinity levels, consequently affect the survival of sea urchin sperm. The survival of sea urchin sperm is important to observe because the sea urchin is an external fertilizing organism resulting in the sperm coming in contact with sea water. The hypothesis is that the survival of sperm will be negatively affected by drastic changes in salinity because Lytechinus variegatus is stenohaline, an organism that reacts harshly to changes in salinity. Salinity levels of 28‰ (parts per thousand), 33‰, 33‰ at pH 6, 38‰, and 43‰ were used in 2.5 min long tests conducted on the survival of sperm. All dead sperm attached to the bottom of the petri dish because of an adhesive protamine sulfate coating, and the sperm was then counted and analysed. The positive control showed the highest percentage of sperm death. Our data showed that only 43‰ had a significantly positive effect on sperm survival, therefore we can conclude changes in salinity do not have a significantly negative effect on the survival of L. variegatus sperm.
Introduction
Due to the developing consequences of greenhouse gas concentrations over the past 50 years, the Earth’s troposphere has increased 2-3o C (Durack and Wijffels, 2010). With this increase, there has been an intensification of the global hydrological cycle (Durack and Wijffels, 2010). Significant salinity changes have been detected in areas where there is an amplified hydrological cycle resulting in a positive increase in salinity levels of approximately 8% for each 1o C increase in water temperature (Durack et al., 2012). If this trend continues, the expected 2-3o C future increase in tropospheric temperature would yield an imminent ocean salinity change of up to +24% (Durack et al., 2012). These resulting salinity concentrations will have a significant effect on the organisms that inhabit the corresponding environments. Salinity levels are an integral factor that affects the survival and reproduction of various marine organisms. L. variegatus is a sea urchin found in the southern region of the eastern United States. This species represents a key herbivore that inhabits a wide range of aquatic habitats including sand flats and seagrass beds (Valentine & Heck, 1999). Spawning of L. variegatus includes patterns that are influenced by environmental conditions and geographical location (Lawrence, 1975). L. variegatus can exhibit moderate eurythermal behavior and can adapt to mild seasonal temperature for short periods of time (Lawrence, 1975). However, chronic exposure to less than ideal temperatures and salinity levels can prove to be destructive to the organism and the vitality of their gametes (Lawrence, 1975). The survival of gametes is an important aspect of reproduction in external fertilizing organisms, which raises the question of sperm survival rates within various levels of salinity. As global warming continues to contribute to environmental change, salinity levels of the ocean and other bodies of water intensify. Due to the predicted 24% increase in ocean salinity levels, the viability of the sperm is expected to be negatively affected (Durack et al., 2012; Fong et al., 1995). These effects can lead to a decreased number of urchins being fertilized, which can lead to a decrease in the population. Knowing if salinity has a negative effect on sperm survival, and to what degree, would provide invaluable information regarding the prevention and/or treatment of the urchin sperm if the salinity is to change. There have been previous experiments that tested the sperm motility and rate of fertilization for various invertebrates with respect to pH, temperature and salinity. These demonstrated that decreased pH levels, increased salinity levels, and harsh immediate change in temperature had negative effects on sperm motility and fertilization rates (Carballeira et al., 2011; Fong et al., 1995; Morita et al., 2010). With ranges from noticeably reduced motility to a complete inhibition of motility for change in salinity to 1.75‰ (parts per thousand) and 3.5‰ or 7.0‰, respectively, Dreissena polymorpha demonstrated a significant response to a drastic change in salinity (Fong et al., 1995). However, there yet to be research conducted on the sperm vitality, the capability to be physically active, of the L. variegatus sea urchin under different salinity levels. Different research indicates that changes in ocean salinity will negatively affect some species sperm vitality and movement capabilities (Fong et al., 1995). In the current study, we examined the effect of changes in salinity on the survival of L. variegatus sperm. It is unknown how the sperm vitality of sea urchins, specifically L. variegatus, are affected by changes in salinity. It is expected that the vitality of L. variegatus sperm will be negatively affected by changes in salinity because L. variegatus is considered stenohaline, meaning the species reacts harshly to severe variations in salinity. We predicted that, when salinity levels rise or fall, the sperm vitality of L. variegatus will fall below normal/functioning levels.
Materials & Methods
A tank filled with artificial sea water of 33‰ was used to house the sea urchins for the entirety of the experiment. The sea water used was kept at 21°C, 7.86 pH, and 33‰. Salinity test levels of 28, 33, 38, and 43‰ were created using an original dilution of 43‰. The gradient for the salinity levels was obtained from a similar study of sea urchins (Saco-Álvarez et al., 2010). Addition of Instant Ocean to the stock artificial seawater allowed the creation of 43‰ seawater, the other levels of seawater salinity were obtained by reducing the salinity of the 43‰ stock. Solutions of each salinity were produced and kept in separate beakers for testing. A separate stock solution of 33‰ with a pH of 6 was produced to be used as a positive control (Saco-Álvarez et al., 2010). The artificial seawater was made more acidic by using HCl. A pH outside of the preferred ~8 pH would result in the decrease of sperm survival. Spawning of the Sea Urchins Sea urchins were purchased from Carolina®, Cat# 162500. The sea urchins were of the species L. variegatus, known to occupy southern tropical regions of the Atlantic Ocean (Moore et al., 1963). The L. variegatus is best suited for warmer waters, resulting in constant care for the organisms. One sea urchin was taken from the tank and wrapped in paper towels. The sea urchin was shaken rapidly to ensure no sea water was left on the organism. In order for the gametes to be collected, the organism must be dry. If the sperm mixes with the seawater, all of the energy (ATP) within each sperm will be activated and used up before analysis. Gametes were obtained by the injection of 2 mL 0.5M KCl roughly 1 cm deep into the perioral membrane of the sea urchin (His et al.,1999). The sea urchin was injected three times. After the injection, gametes release from the top of the sea urchin. Once gametes start to release, they were transferred from the sea urchin into sterile eppendorf tubes via a micropipette (His et al.,1999). Different eppendorf tubes were used for gametes of different sea urchins and stored in a refrigerator at a temperature of 4°C (Carballeira et al., 2011). Salinity Test on Sea Urchin Sperm Sperm was diluted to a ratio of 1µl of sperm:10µl of water for the salinity tests. This highly concentrated suspension was reduced in concentration when added to the petri dish. It is important for the final concentration to be 1µl of sperm:4000µl of water since this is a concentration at which sperm can be efficiently counted from under a microscope. The final dilution ratio is reached when the highly concentrated sperm suspension is added to the 12 ml of water which is in each petri dish. Each petri dishes bottom was coated with protamine sulfate, which has adhesive properties. The sticky petri dish bottoms would capture any dead sperm, since the dead sperm will sink to the bottom of the petri dish while the living sperm swim throughout the treatment water. Six petri dishes were used, all petri dishes were provided by Dr. Nam’s lab at Rutgers University,; 28‰, 33‰, 38‰, 43‰, negative control (33‰ and 6 pH), and the procedural control (33‰). The sample size at each treatment level is three. Each petri dish contained 12 mL of the respective treatment. A dilution of 18µl of sperm in 180µl of water was created and then rapidly pipetted into each treatment. Three microliters were added into the center of each petri dish, and the sperm stayed in the petri dish for 2.5 minutes. The 12 mL of water was taken out after 2.5 minutes from each petri dish except from the procedural control. A time of 5 minutes of exposure was used in a previous experiment (Dinnel et al., 1981) but after conducting pilot tests on the life span of the sperm that was collected under conditions in our lab it was decided that treatment time must be shortened to the 2.5 minutes used. This time was determined after the life of our sperm was found to be 11- 12 minutes. Through preliminary testing it was determined that at 2.5 minutes the sperm was affected by treatment. After the treatments had been removed, 12 mL of the matching treatment group was added back to each treated petri dish. Each treatment was used with the sperm collected from three sea urchins. Each petri dish was placed under a 400x microscope for sperm count. A circular grid template was made and used for each petri dish, the circular grid having three zones (rings) and eight boxes per zone. The sperm present in each box was counted for, and the average of each zone was found. Using the averages of each zone, the total number of sperm on the petri dish was found. The number of sperm on each of the treatment petri dishes was compared to the procedural control resulting in the percent of dead sperm present on the petri dish. Sperm Distribution The circular grid template has four boxes (three boxes if not including the center) going from the center to the edge in eight directions. The counting of sperm in each box allows for the understanding of the dXispersion of sperm after it is added to the treatment. The center shows the amount of sperm present at the point the sperm was added. Figure 1 shows the circular grid template and explains its dimensions. Each zone is in a ring shape and each zone moves further from the center. The size of the boxes are different but this is irrelevant because the field of view of the 400x microscope will always be smaller than the size of the box for each zone. The number of sperm in each box throughout the three replications will be averaged and used to determine if differences in the sperm distribution are significant.
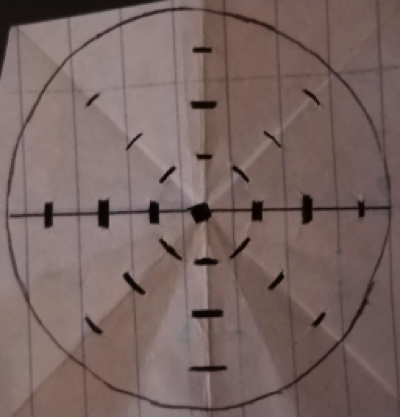
Figure 1. The grid template is 5.5 cm diameter circle, the same size as the petri dishes used. A box was made in the center. The eight boxes immediately surrounding the center box comprise Zone 1. The eight boxes immediately surrounding Zone 1, comprise Zone 2. The eight boxes immediately surrounding Zone 2, comprise Zone 3. From the center box each box in Zone 1 is 0.75 cm in distance. Zone 2 boxes are 1.5 cm away from the center box and Zone 3 boxes are 2.25 cm away from the center box.
Statistical analysis
The sperm tested was collected from three sea urchins of the same species, coming from the same provider. Sperm from each sea urchin was tested on five treatments with an additional procedural control for comparison. The statistical test used for the comparison between the negative control, 33‰, and other treatments was a T-test since a Shapiro-Wilk normality test was not violated. The statistical test ran on the sperm distribution data was a Chi-Squared test after it was determined the Shapiro-Wilk normality test was violated.
Results
As expected we found the highest percent of dead sperm at the 33‰ at pH 6 and the positive control at 74.8% (Fig. 2). The next highest is the 33‰ negative control at 62.0%, then the 38‰ treatment group at 60.9%, then the 28‰ treatment group at 48.1%, and finally the 43‰ treatment group at 15.7%. Sperm mortality did not vary significantly for L. variegatus exposed to salinities under 43‰ (Fig. 2, two sample t-test, for 28‰ p-value = 0.50; for 33‰ 6 pH p-value = 0.70; for 38‰ p-value = 1.0). There was significantly lower sperm mortality when L. variegatus sperm were exposed to 43‰, the highest salinity treatment (Fig. 2, two sample t-test, for 43‰ p-value = 0.02). Next, we measured the mobility of the sperm. There were statistically significant differences in the data found from distribution amongst zones between 33‰ and 43‰. Statistical tests show that the data found between the control and treatment group at each zone was significantly different (Fig. 3, Chi-Squared Test: p-value < 0.05). There are differences in the two treatments which can be seen at the Center, Zone 2, and Zone 3, with higher dead sperm concentration present in the outer rings at higher salinities, the data and test show there is a significant trend.

Figure 2. The effect of salinity on the survival of L. variegatus sperm. Sperm death percentage of L. variegatus was recorded after a 2.5 minute period. Percent sperm death in L. variegatus in several saline conditions. Values are mean ± SE, n =3. Asterisk over bar indicates p-value < .05.

Figure 3. The effect of salinity on the dispersion of L. variegatus sperm. Percent sperm death in each zone; Center, Zone 1, Zone 2 and Zone 3 displayed for 33‰ and 43‰. Values are mean ± SE, n=3. Overall significance, p < 0.05. Discussion According to Figure 2, L. variegatus sperm exhibited no observable trend when exposed to various levels of salinities, exhibiting lower percent sperm death at the highest level of salinity. The results of our experiment suggest that sperm is not negatively affected by higher and lower levels of salinity. There was no significant difference between the 33‰ (negative control) and 28‰, 33‰ at pH 6, and 38‰ (p > 0.05). The positive control was expected to have a increased change in percent sperm death, there may have been an error in the execution of the experiment (Morita et al., 2010). During many steps; the making of sperm to water concentrations, the amount of time before taking out the treatment, and the amount of time the sperm was kept before doing the experiment could all have an effect on sperm death rates. Each step can be refined and made more exact in timing and performance in an effort to reduce error in the future. There was a significant difference between the 43‰ and 33‰ (negative control) (p < 0.05), but is the opposite of the result expected. There was expected to be a much higher percent death in the 43‰ treatment group, but the experiment yielded significantly lower percent death than the negative control. One reason this may be the case is if osmotic shock is the main factor behind this significant change. Osmotic shock could have led to the insignificance of the other treatment groups of 28‰ and 33‰ (Curry and Watson, 1994). Exposing the sperm from 33‰ directly to the other treatment groups was an experimental flaw. The original hypothesis cannot be accepted because 43‰ did not yield more dead sperm while the normal of 33‰ did. The null hypothesis that the survival of sperm will be positively affected by drastic changes in salinity could be accepted according to the data collected. The distribution of sperm shown in Figure 3 follows similar trends in the treatments group of 28‰ and the positive and negative controls with very low concentrations of sperm in Zones 2 and 3. The treatment groups of 38‰ and 43‰ show a slightly different trend, with slightly higher concentrations among Zones 2 and 3. Because there is a slight difference, this may be an experiment that should be reproduced in a slightly different manner to determine if high salinity does in fact change the sperm distribution of L. variegatus sperm. If the distribution data is looked at along with the sperm survival data there is a relationship between the sperm distributing further in the salinities when the least amount of sperm death is observed. Since there is more sperm alive at 43‰ it would be safe to think that the sperm has more time to travel. This relationship does support the null hypothesis since it shows the sperm is not only surviving at high salinities, the sperm is able to disperse further. This is the first paper to determine the vitality of sperm in potential future marine environments of L. variegatus. To get a more accurate reading of the total percentage of dead sperm, future studies can use serial transfers into slightly higher or lower salinities to prevent osmotic shock. Sperm analysis would be appropriate for determining whether varying environmental factors have negative effects that could critically affect the overall well-being of the species. Sperm vitality is fundamental to the success of the reproduction of the L. purpuratus, and this analysis could become an essential method of research on these species as the environment continues to be compromised. Acknowledgements We would like to thank Dr. Jongmin Nam for help and support in the conducting of our experiment.
References
Carballeira, C., Martín-Díaz, L., and DelValls, T.A. (2011). Influence of salinity on fertilization and larval development toxicity tests with two species of sea urchin. Marine Environmental Research 72, 196–203.
Curry, M.R., and Watson, P.F. (1994). Osmotic Effects on Ram and Human Sperm Membranes in Relation to Thawing Injury. Cryobiology 31, 39–46.
Dinnel, P.A., Stober, Q.J., and DiJulio, D.H. (1981). Sea urchin sperm bioassay for sewage and chlorinated seawater and its relation to fish bioassays. Marine Environmental Research 5 29–39.
Durack, P.J., and Wijffels, S.E. (2010). Fifty-Year Trends in Global Ocean Salinities and Their Relationship to Broad-Scale Warming. Journal of Climate 23, 4342–4362.
Durack, P.J., Wijffels, S.E., and Matear, R.J. (2012). Ocean salinities reveal strong global water cycle intensification during 1950 to 2000. Science 336, 455–458.
Fong, P.P., Kyozuka, K., Duncan, J., Rynkowski, S., Mekasha, D., and Ram, J.L. (1995). The effect of salinity and temperature on spawning and fertilization in the zebra mussel Dreissena polymorpha (Pallas) from North America. The Biological Bulletin 189, 320–329.
His, E., Heyvang, I., Geffard, O., de Montaudouin, X., 1999. A comparison between oyster (Crassostrea gigas) and sea urchin (Paracentrotus lividus) larval bioassays for toxicological studies. Water Research 33 1706e1718.
Morita, M., Suwa, R., Iguchi, A., Nakamura, M., Shimada, K., Sakai, K., and Suzuki, A. (2010). Ocean acidification reduces sperm flagellar motility in broadcast spawning reef invertebrates. Zygote 18, 103–107.
Saco-Álvarez, L., Durán, I., Ignacio Lorenzo, J., and Beiras, R. (2010). Methodological basis for the optimization of a marine sea-urchin embryo test (SET) for the ecological assessment of coastal water quality. Ecotoxicology and Environmental Safety 73, 491–499.
Valentine, J.F., Heck Jr, K.L., Kirsch, K.D., and Webb, D. (2000). Role of sea urchin Lytechinus variegatus grazing in regulating subtropical turtlegrass Thalassia testudinum meadows in the Florida Keys (USA). Marine Ecology Progress Series 213–228.
Journal of Biological Sciences at Rutgers Camden (JBS) is licensed under a Creative Commons Attribution-NonCommercial-ShareAlike 4.0 International License